Volume 4 - Year 2021- Pages 68-77
DOI: 10.11159/ijci.2021.010
Workability and Early-Age Strength of Recycled Aggregate Concrete Incorporating Basalt Fibers
Shahrukh Shoaib1, Hilal El-Hassan1, Bilal El-Ariss1, Tamer El-Maaddawy1
1United Arab Emirates University, Department of Civil and Environmental Engineering
P.O. Box 15551, Al Ain, United Arab Emirates
201890010@uaeu.ac.ae; helhassan@uaeu.ac.ae; bilal.elariss@uaeu.ac.ae; tamer.maaddawy@uaeu.ac.ae
Abstract - This research investigates the effect of basalt fibers (BF) on the workability and early-age compressive and splitting tensile strengths of concrete made with 100% recycled concrete aggregates (RCA). The target concrete compressive strengths were 30, 45, and 60 MPa, whereas the basalt fibers had a length of either 20 or 43 mm. The addition of BF significantly decreased the workability, slightly improved the compressive strength, and remarkably increased the splitting tensile strength of the RCA-based concrete. The compressive strengths of the RCA-based concrete with different BF lengths and volume fractions were insignificantly different. The original compressive strength of the natural aggregate (NA)-based concrete was not fully restored, irrespective of the BF length and volume fraction. Basalt fibers had a more pronounced effect on improving the splitting tensile strength rather than the compressive strength. The original splitting tensile strength of the NA-based concrete was fully restored in most of the cases. Basalt fibers with a length of 43 mm were more effective in improving the splitting tensile strength of the RCA-based concrete than those having a length of 20 mm.
Keywords: Basalt fibers, recycled concrete aggregates, workability, compressive strength, splitting tensile strength.
© Copyright 2021 Authors - This is an Open Access article published under the Creative Commons Attribution License terms. Unrestricted use, distribution, and reproduction in any medium are permitted, provided the original work is properly cited.
Date Received: 2020-12-18
Date Accepted: 2020-12-22
Date Published: 2021-04-06
1. Introduction
The rapid increase in the human population demands infrastructure development, instigating a need for more construction materials [1], [2]. Of these materials, concrete is one of the most commonly used [3]. It is typically made of 20-25% binding paste, i.e. cement and water, and 75-80% filler, i.e. aggregates. These aggregates are created through the processing of natural stone. Thus, their use in concrete results in the depletion of non-renewable natural resources [4], [5]. Therefore, it is essential to find a material that can replace natural aggregates in concrete without significantly affecting its mechanical and durability characteristics.
Concrete structures are demolished after producing a huge amount of construction and demolition waste (CDW) [6], [7] . Generally, CDW is disposed of into landfills. Yet, with limited land, this technique is becoming less desirable. Moreover, the leeching of hazardous materials from CDW results in environmental pollution [8], [9]. Therefore, it is necessary to provide a novel environment-friendly waste management technique to dispose of the CDW. In one method, CDW was processed and successfully converted into recycled concrete aggregates (RCA) [10]. However, concrete incorporating RCA possessed inferior mechanical properties and durability characteristics compared to those made with natural aggregates [11]–[20]. Kachouh et al. [21] noticed 18, 41, and 47% reductions in compressive strength at RCA replacement of 30, 70, and 100%, respectively. Flexural strength of concrete was reduced by 16, 46, and 51% at similar RCA replacement [22]. Due to such inferior performance, the adoption of RCA by the construction industry instead of natural aggregates is hindered. To counteract the negative impact of RCA on the properties of concrete, fiber reinforcement was utilized [21]–[23]. The addition of 2% steel fiber (SF) improved the compressive strength of 100% RCA-concrete by 9% [21]. Though, these added SF are susceptible to corrosion [24]. Accordingly, it is preferred to use non-metallic fibers that are not corrodible, including basalt fibers (BF). This paper examines the effect of adding BF on the workability and early-age compressive and splitting tensile strengths of concrete made with 100% RCA.
2. Related Work
There is limited literature available on the effect of incorporating BF in the mixture on the mechanical properties of concrete made with RCA. Wang et al [25] investigated the combined influence of micro-BF and nano-silica on the mechanical properties of concrete incorporating 100% RCA. Basalt fibers of 32 mm length and 15 µm diameter were used. Their addition ranged between 0 and 3 kgm-3, whereby the highest fiber content improved the compressive strength by 3.82%. Additionally, Fang et al. [26] studied the mechanical properties of 50% RCA concrete reinforced with 18-mm long BF. The elastic modulus of concrete made with 50% RCA improved by 1.29% at BF volume fraction of 0.2%. Moreover, Dong et al. [27] evaluated the effect of BF on the mechanical properties of 50-100% RCA concrete. Basalt fibers were added in the range of 0 to 4 kgm-3. Results showed that the addition of 2 kgm-3 BF improved the 28-day cube compressive strength of 100% RCA-concrete by 10.8%.
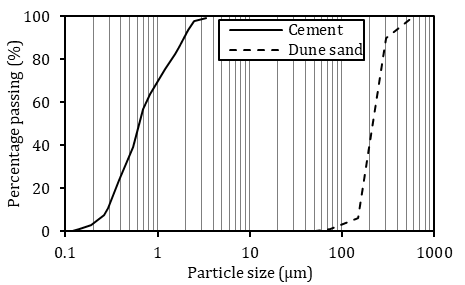
Table 1. Chemical composition of cement and dune sand
Compound | Cement | Dune sand |
SiO2 (%) | 19.9 | 64.9 |
Al2O3 (%) | 4.9 | 3 |
Fe2O3 (%) | 2.3 | 0.7 |
CaO (%) | 63.2 | 14.1 |
MgO (%) | 2.5 | 1.3 |
Na2O (%) | 0.4 | 0.4 |
K2O (%) | 0.7 | 1.1 |
LOI (%) | 2.6 | - |
Others (%) | 3.5 | 14.5 |
3. Materials and Methods
3. 1. Materials
ASTM Type I cement, natural aggregates, recycled concrete aggregates, dune sand, and tap water were used in the preparation of concrete mixes. The gradation curves of dune sand and cement are shown in Figure 1. The chemical composition of dune sand (DS) and cement was determined by X-ray fluorescence (XRF) analysis, as shown in Table 1. The different compounds found in cement and dune sand are shown in the X-ray diffraction (XRD) pattern of Figure 2.
Coarse aggregates were natural aggregates (NA) and RCA. The nominal maximum size (NMS) of NA was 19 mm whereas that of RCA was 25 mm. The NA were obtained from a local quarry, while the RCA were acquired from a CDW recycling plant. In turn, desert dune sand served as fine aggregates. Table 2 shows the physical characteristics of the aggregates used. The water absorption of RCA was more than that of NA, whereas its resistance to abrasion was lower. The physical appearance of NA, RCA, and dune sand is shown in Figure 3.
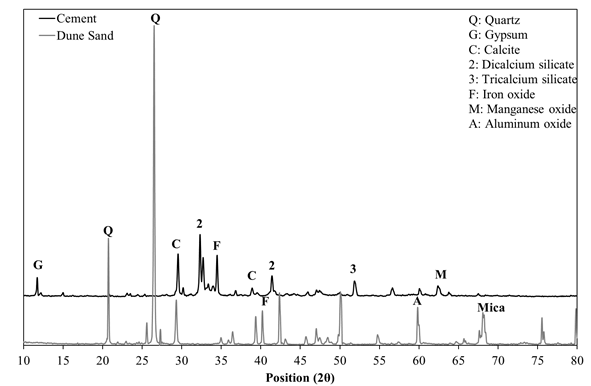
Table 2. Physical properties of coarse and fine aggregates
Property | Unit | Standard Test | NA | RCA | DS |
Surface area | cm2/g | ASTM C136 [28] | 2.49 | 2.50 | 116.8 |
Soundness (MgSO4) | % | ASTM C88 [29] | 1.20 | 2.78 | - |
Specific gravity | – | ASTM C127 [30] | 2.82 | 2.63 | 2.77 |
Dry-rodded density | kg/m3 | ASTM C29 [31] | 1635 | 1563 | 1663 |
Los Angeles abrasion | % | ASTM C131 [32] | 16.0 | 32.6 | - |
Absorption | % | ASTM C127 [30] | 0.22 | 6.63 | - |
Fineness modulus | – | ASTM C136 [28] | 6.82 | 7.44 | 1.45 |
Basalt fibers of 20 and 43 mm length were used in the preparation of the concrete mixes. The properties of the BF, as provided by the manufacturer, are listed in Table 3. The physical appearance of BF is shown in Figure 4.
A polycarboxylic ether polymer-based superplasticizer (SP) was added to improve the workability of the concrete mix without compromising the mechanical properties. The target slump was set to 150 mm.
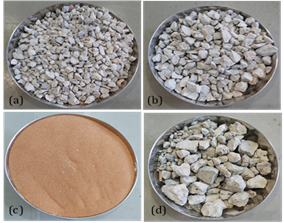
Table 3. Properties of basalt fibers [33]
Diameter (mm) | Tensile strength (MPa) | Elastic modulus (GPa) | Density (gcm-3) |
0.72 | > 900 | 44 | 2.1 |
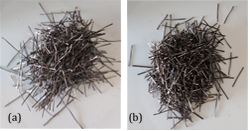
3. 2. Mixture Proportions
Three different mixes were designed based on ACI 211.1 [34] to attain target compressive strengths of 30, 45, and 60 MPa and a slump of at least 150 mm. Concrete mixes prepared with NA only served as a benchmark. Table 4 presents the mixture proportions of each concrete mix. Concrete mixes were labelled as xRyBFz/w, where x is target compressive strength (MPa), y is the RCA replacement percentage (%), z is the volume fraction of basalt fibers (%), and w indicates the length of BF (in mm). For instance, 45R100BF1.5/43 represents a 45-MPa concrete prepared with 100% RCA and 1.5% volume fraction of BF having a length 43 mm.
3. 3. Sample Preparation
Concrete mixes were prepared in the laboratory at an ambient temperature of 25+2°C and a relative humidity of 50+5 %. Coarse aggregates (NA or RCA) were first brought up to saturated surface dry (SSD) condition to accommodate for their water absorption. The SSD aggregates and dune sand were then added to the mixer and mixed for 3 minutes. The cement and water were subsequently added, and mixing continued for 3 more minutes. Basalt fibers were added at the end of mixing to avoid the breakage of fibers, as shown in Figure 5. For each mix, three replicate concrete specimens were cast and covered with plastic sheet for 24 hours, after which the specimens were removed from the moulds and cured in water for 7 days.
Table 4. Mix proportions of concrete mixes
Mix Designation | Mass (kg/m3) | ||||||
Cement | Sand | NA | RCA | Water | SP | BF | |
30R0BF0 | 470 | 570 | 1130 | 0 | 230 | 0.00 | 0.0 |
30R100BF0 | 470 | 570 | 0 | 1130 | 230 | 0.00 | 0.0 |
30R100BF0.5/43 | 470 | 570 | 0 | 1130 | 230 | 0.00 | 10.5 |
30R100BF1/43 | 470 | 570 | 0 | 1130 | 230 | 0.00 | 21.0 |
30R100BF1.5/43 | 470 | 570 | 0 | 1130 | 230 | 0.00 | 31.5 |
30R100BF0.5/20 | 470 | 570 | 0 | 1130 | 230 | 0.00 | 10.5 |
30R100BF1/20 | 470 | 570 | 0 | 1130 | 230 | 0.00 | 21.0 |
30R100BF1.5/20 | 470 | 570 | 0 | 1130 | 230 | 0.00 | 31.5 |
30R100BF2/20 | 470 | 570 | 0 | 1130 | 230 | 0.00 | 42.0 |
30R100BF3/20 | 470 | 570 | 0 | 1130 | 230 | 0.00 | 63.0 |
45R0BF0 | 567 | 543 | 1100 | 0 | 216 | 0.62 | 0.0 |
45R100BF0 | 567 | 543 | 0 | 1100 | 216 | 0.62 | 0.0 |
45R100BF0.5/43 | 567 | 543 | 0 | 1100 | 216 | 0.62 | 10.5 |
45R100BF1/43 | 567 | 543 | 0 | 1100 | 216 | 0.62 | 21.0 |
45R100BF1.5/43 | 567 | 543 | 0 | 1100 | 216 | 0.62 | 31.5 |
45R100BF0.5/20 | 567 | 543 | 0 | 1100 | 216 | 0.62 | 10.5 |
45R100BF1/20 | 567 | 543 | 0 | 1100 | 216 | 0.62 | 21.0 |
45R100BF1.5/20 | 567 | 543 | 0 | 1100 | 216 | 0.62 | 31.5 |
45R100BF2/20 | 567 | 543 | 0 | 1100 | 216 | 0.62 | 42.0 |
45R100BF3/20 | 567 | 543 | 0 | 1100 | 216 | 0.62 | 63.0 |
60R0BF0 | 617 | 513 | 1079 | 0 | 216 | 0.92 | 0.0 |
60R100BF0 | 617 | 513 | 0 | 1079 | 216 | 0.92 | 0.0 |
60R100BF0.5/43 | 617 | 513 | 0 | 1079 | 216 | 0.92 | 10.5 |
60R100BF1/43 | 617 | 513 | 0 | 1079 | 216 | 0.92 | 21.0 |
60R100BF1.5/43 | 617 | 513 | 0 | 1079 | 216 | 0.92 | 31.5 |
60R100BF0.5/20 | 617 | 513 | 0 | 1079 | 216 | 0.92 | 10.5 |
60R100BF1/20 | 617 | 513 | 0 | 1079 | 216 | 0.92 | 21.0 |
60R100BF1.5/20 | 617 | 513 | 0 | 1079 | 216 | 0.92 | 31.5 |
60R100BF2/20 | 617 | 513 | 0 | 1079 | 216 | 0.92 | 42.0 |
60R100BF3/20 | 617 | 513 | 0 | 1079 | 216 | 0.92 | 63.0 |
3. 4. Performance Evaluation
More than 150 samples were tested in the laboratory to determine the workability and early-age mechanical properties of the concrete. The workability of fresh concrete was measured using the slump cone test as per ASTM C143 [35]. Cubes of 100 mm size and cylinders of 100 mm x 200 mm (diameter x height) were used to determine the compressive and tensile strengths as per BS EN 12390-3 [36] and ASTM C496 [37], respectively. For both tests, the load was applied using a 2000-kN compression testing machine at respective loading rate of 7 and 1 kN/s. The average of three concrete replicate samples was used in the analysis of compressive and splitting tensile strengths.
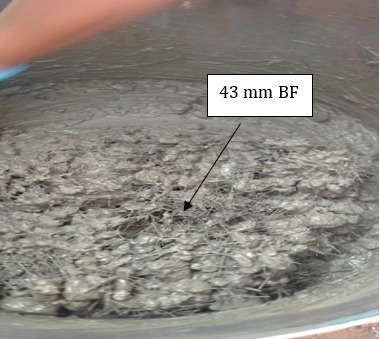
4. Results and Discussion
4. 1. Slump
The workability of concrete was characterized by the slump test. Figure 6 demonstrates the workability of different concrete mixes. The horizontal lines represent the workability of control mixes made with NA and 0% BF and having target compressive strengths of 30, 45, and 60 MPa. The slump values of these control mixes were 155, 215, and 220 mm, respectively. The replacement of NA by 100% RCA did not have an impact on the slump of the 30- and 45-MPa concrete. However, the slump decreased by 16% for 60-MPa 100% RCA-concrete. Apparently, the irregular shape and rough surface texture of RCA became more influential at higher strength [38].
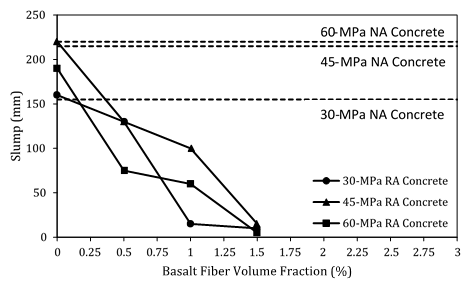
Figure 6 also shows the effect of 43-mm basalt fiber addition on the workability, i.e. slump. For RCA concrete with a target strength of 30 MPa, the addition of 0.5, 1, and 1.5% 43-mm BF, by volume, resulted in slump of 130, 15, and 10 mm, representing reductions of 16, 90, and 94%, respectively. Contrarily, BF incorporation into RCA concrete designed to attain strengths of 45 and 60 MPa led to decreases of slump up to 93 and 98, respectively.
The use of shorter, 20 mm, basalt fibers presented a similar trend, as shown in Figure 7. RCA concrete with 30, 45, and 60 MPa design strength experienced up to 48, 77, and 73% loss in slump, respectively. Clearly, the addition of basalt fibers reduced the workability with higher volume fractions and longer fibers having a more pronounced adverse impact.
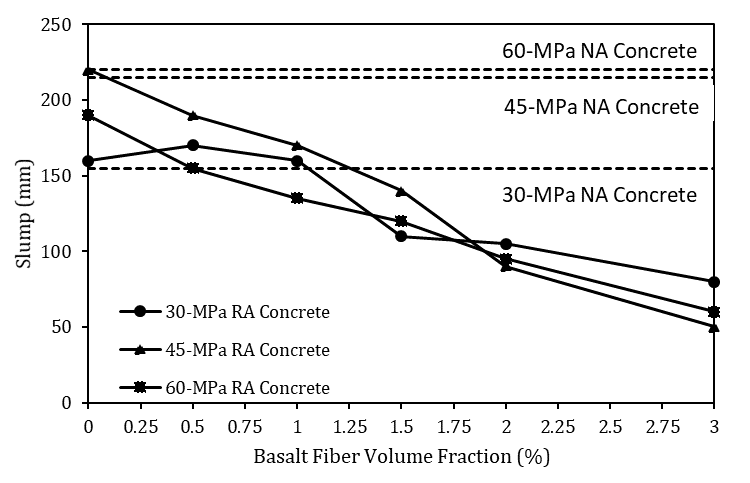
4. 2. Compressive Strength
The 7-day compressive strength of concrete mixes made with different RCA replacement and BF volume fractions is shown in Figure 8. The mix designated as “CON” represents the control mix made with 100% NA and 0% BF. Regardless of the target strength, the replacement of NA by 100% RCA decreased the concrete compressive strength. In fact, the compressive strength of concrete designed to attain 30, 45, and 60 MPa strength decreased by 49, 47, and 58%, respectively. Clearly, the impact of RCA replacement is more noticeable in concrete with higher design strength.
The addition of BF generally improved the compressive strength of 100% RCA-concrete. Yet, it is worth noting that mixes incorporating 1.5% of 43-mm had limited workability and could not be compacted into samples for testing. Detailed strength values and percent change due to basalt fiber addition are shown in Table 5. For 30-MPa design strength concrete, the addition of 0.5 and 1% of 43-mm BF enhanced the compressive strength by 27 and 21%, respectively. This shows that volume fractions beyond 0.5% for the 43-mm BF do not provide any additional strength. Conversely, adding 20-mm BF by up to 3%, by volume, improved compressive strength of 100% RCA-concrete by up to 29%. Although, the addition of BF improved the compressive strength of 100% RCA-concrete, it is worth finding the strength retention as percentage of NA-based concrete, as shown in the last column of Table 5. The strength retention of 100% RCA-concrete reinforced with 43- and 20-mm BF at volume fraction of up to 1 and 3% could retain up to 65 and 70% of the compressive strength of the control mix, respectively.
For 45-MPa concrete, the addition of 43- and 20-mm BF at volume fraction of up to 1 and 3% resulted in up to 16% improvement in compressive strength for 100% RCA-concrete. The retention of the compressive strength retention of concrete reinforced with 43-mm BF ranged between 61 and 62%. Counterparts made with 20-mm BF could retain between 57 and 62% of the compressive strength of the NA-based concrete.
Table 5. Compressive strength of BF-reinforced concrete
Mix Designation | Avg. fcu (MPa) | Increase in fcu compared to RCA concrete (%) | Retained fcu of percent of NA Concrete (%) |
30R0BF0 | 33.4 | - | - |
30R100BF0 | 17.2 | - | - |
30R100BF0.5/43 | 21.9 | 27 | 65 |
30R100BF1/43 | 20.8 | 21 | 62 |
30R100BF0.5/20 | 23.5 | 37 | 70 |
30R100BF1/20 | 18.9 | 10 | 58 |
30R100BF1.5/20 | 22.2 | 29 | 66 |
30R100BF2/20 | 21.5 | 25 | 64 |
30R100BF3/20 | 20.1 | 17 | 60 |
45R0BF0 | 44.9 | - | - |
45R100BF0 | 23.9 | - | - |
45R100BF0.5/43 | 27.2 | 14 | 61 |
45R100BF1/43 | 27.7 | 16 | 62 |
45R100BF0.5/20 | 27.2 | 14 | 61 |
45R100BF1/20 | 27.7 | 16 | 62 |
45R100BF1.5/20 | 27.3 | 14 | 61 |
45R100BF2/20 | 27.5 | 15 | 61 |
45R100BF3/20 | 25.7 | 8 | 57 |
60R0BF0 | 52.7 | - | - |
60R100BF0 | 22.1 | - | - |
60R100BF0.5/43 | 27.6 | 25 | 52 |
60R100BF1/43 | 30.8 | 39 | 58 |
60R100BF0.5/20 | 24.5 | 11 | 46 |
60R100BF1/20 | 25.3 | 14 | 48 |
60R100BF1.5/20 | 29.1 | 32 | 55 |
60R100BF2/20 | 29.3 | 33 | 56 |
60R100BF3/20 | 27.0 | 22 | 51 |
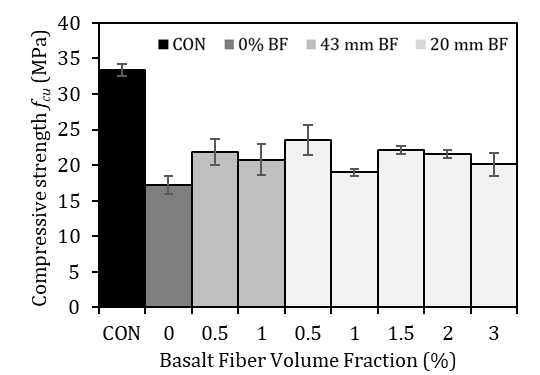
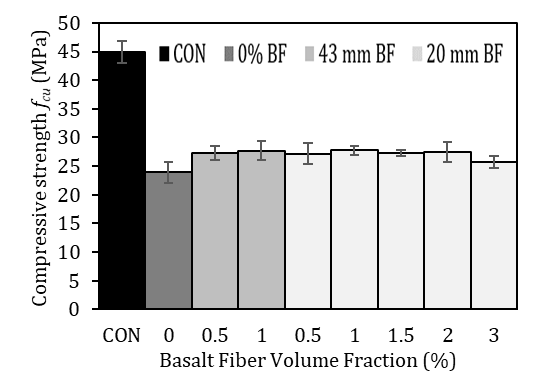
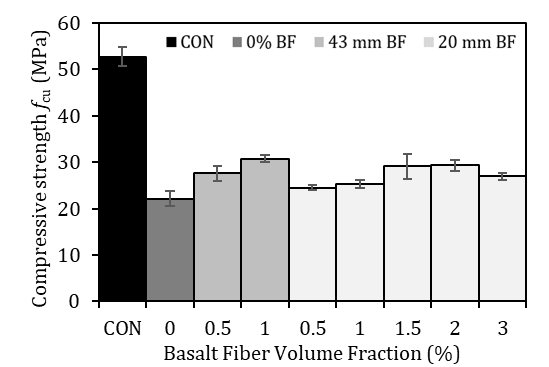
Considering the 60-MPa concrete, the compressive strength of 100% RCA-concrete improved by 25 and 39% through the addition of 43-mm BF at volume fractions of 0.5 and 1%, respectively. However, the addition of 20-mm BF at volume fraction of 0.5-3% improved the compressive strength by 11-33%. In terms of strength retention as percent of NA-based concrete, RCA-concrete reinforced with 43-mm BF could retain up 56% of the strength. Mixes reinforced with 20-mm BF had retention values up to 56%.
From the obtained results, it can be noticed that the addition of basalt fibers increased the compressive strength of RCA-concrete, regardless of BF length. In fact, the strength improvement was similar upon adding 20 or 43 mm BF. Similar findings can be perceived for strength retention. Yet, it is worth noting that the incorporation of 20- and 43-mm basalt fibers had more pronounced impact at lower and higher design strengths, respectively.
As noted earlier, three replicate samples were prepared and tested to obtain the average compressive strength. The accuracy of the obtained results is evaluated by determining the standard deviation and coefficient of variation. Table 6 shows that the standard deviation ranged between 0.5 and 2.7 MPa, while the coefficient of variation was in the range of 1.9-9.3% (average of 5%). This shows that the dispersion of test results is relatively low with high repeatability, high precision, and limited uncertainty.
4. 3. Splitting Tensile Strength
The splitting tensile strength of concrete mixes tested at 7 days is shown in Figure 9. Replacement of NA by 100% RCA decreased the splitting tensile strength by 21, 35, and 40% for concrete with design strength of 30, 45, and 60 MPa, respectively. Compared to compressive strength, it is obvious that effect of RCA replacement is less detrimental on splitting tensile strength.
The effect of adding 20- and 43-mm basalt fibers on the splitting tensile strength is examined. Strength values, percent increase due to basalt fiber addition, and strength retention percentage as a function of the NA-based values are shown in Table 6. Considering the 30-MPa design strength concrete, the addition of 0.5 and 1% BF improved splitting tensile strength of 100% RCA-concrete by 45 and 86%, respectively. In comparison, splitting tensile improved by 36, 25, 42, 30, and 48% when BF was added in respective volume fractions of 0.5, 1, 1.5, 2, and 3%. Furthermore, the strength retention of RCA-concrete mixes incorporating up to 1 and 3% volume fraction of 43- and 20-mm BF was 147 and 117% that of the control mix. Apparently, BF are effective in improving the splitting tensile strength of RCA-concrete. Yet, it is worth mentioning that 43-mm BF were more effective than 20-mm counterparts, owing to their larger length and improved bridging capacity.
For 45-MPa design strength concrete, the splitting tensile strength of RCA-concrete improved by 47 and 109% by the respective addition of 0.5 and 1% of 43-mm BF compared to plain RCA-concrete. Moreover, for 20-mm the maximum splitting tensile strength retention for 43- and 20-mm BF-reinforced RCA-concrete was 135 and 113%, respectively. Similar to concrete with a 30-MPa design strength, longer BF further enhanced the splitting tensile strength.
As for the 60-MPa design strength concrete, the addition of up to 1 and 3% of 43- and 20-mm BF, by volume, increased the splitting tensile strength by up to 116 and 89%, respectively, compared to plain RCA-concrete. With reference to the control mix, the strength retention level could reach up to 132 and 115%.
Table 6. Statistics related to the compressive and splitting tensile strength
fcu | ft | |||
Mix Designation | StDeva (MPa) | COVb (%) | StDev (MPa) | COV (%) |
30R0BF0 | 0.9 | 2.6 | 0.16 | 9.0 |
30R100BF0 | 1.2 | 7.1 | 0.11 | 8.8 |
30R100BF0.5/43 | 1.8 | 8.4 | 0.13 | 6.8 |
30R100BF1/43 | 1.1 | 5.7 | 0.13 | 5.4 |
30R100BF0.5/20 | 2.1 | 9.1 | 0.16 | 9.0 |
30R100BF1/20 | 0.5 | 2.6 | 0.16 | 9.9 |
30R100BF1.5/20 | 0.6 | 2.5 | 0.07 | 3.6 |
30R100BF2/20 | 0.6 | 2.7 | 0.11 | 6.6 |
30R100BF3/20 | 1.6 | 8.1 | 0.13 | 6.7 |
45R0BF0 | 1.9 | 4.3 | 0.08 | 3.7 |
45R100BF0 | 1.8 | 7.7 | 0.15 | 9.9 |
45R100BF0.5/43 | 1.2 | 4.4 | 0.13 | 5.9 |
45R100BF1/43 | 1.7 | 6.2 | 0.19 | 6.0 |
45R100BF0.5/20 | 1.8 | 6.7 | 0.05 | 2.1 |
45R100BF1/20 | 0.8 | 2.7 | 0.10 | 5.0 |
45R100BF1.5/20 | 0.5 | 1.9 | 0.10 | 4.4 |
45R100BF2/20 | 1.8 | 6.4 | 0.07 | 2.8 |
45R100BF3/20 | 1.0 | 4.1 | 0.11 | 4.3 |
60R0BF0 | 2.1 | 4.0 | 0.06 | 2.6 |
60R100BF0 | 1.6 | 7.2 | 0.13 | 8.7 |
60R100BF0.5/43 | 1.6 | 5.7 | 0.23 | 9.5 |
60R100BF1/43 | 0.7 | 2.3 | 0.15 | 4.5 |
60R100BF0.5/20 | 0.5 | 2.1 | 0.17 | 9.8 |
60R100BF1/20 | 0.9 | 3.6 | 0.07 | 4.0 |
60R100BF1.5/20 | 2.7 | 9.3 | 0.19 | 7.8 |
60R100BF2/20 | 1.2 | 4.1 | 0.18 | 7.2 |
60R100BF3/20 | 0.8 | 2.9 | 0.11 | 3.9 |
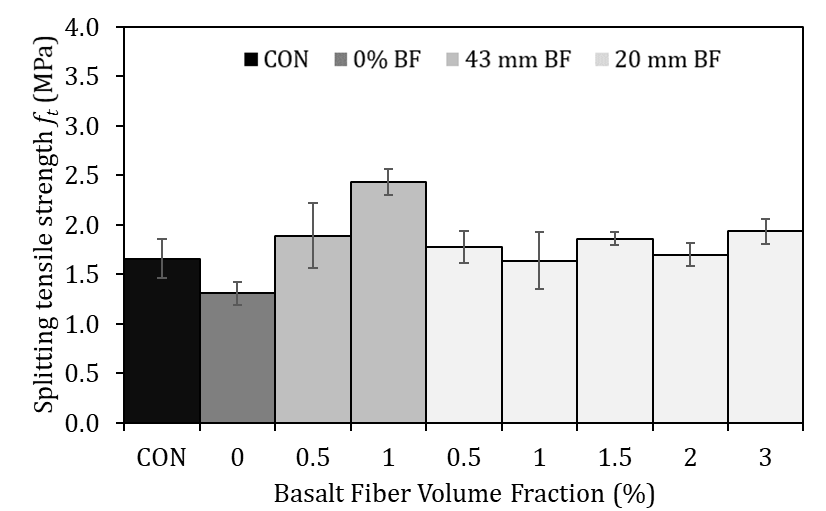
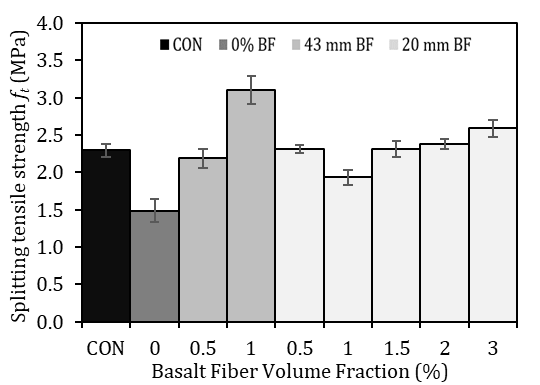
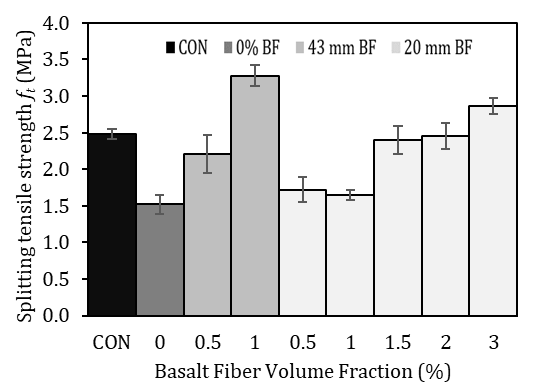
Table 7 depicts the ratio of the splitting tensile strength to the compressive strength. Independent of the design strength, mixes made with 100% RCA had a higher ratio than those made with 100% NA. This provides evidence to the fact that the replacement of NA by RCA was less impactful on the tensile strength than the compressive strength. Furthermore, the incorporation of 43- and 20-mm BF resulted in a higher ratio, signifying its more pronounced effect on the splitting tensile strength. However, it is worth noting that, irrespective of the concrete design strength, the 43-mm BF-reinforced concrete provided a higher ratio than 20-mm counterpart, taking into consideration that the former had a maximum volume fraction of 1% while the that of the latter could reach 3%. Based on the results of this study, it can be concluded that the integration of 43-mm BF in RCA-concrete is preferred over the 20-mm BF for superior early-age compressive and splitting tensile strengths.
To determine the average splitting tensile strength, three replicate samples were prepared and tested. Similar to the compressive strength, the accuracy of the splitting tensile results is assessed using the standard deviation and coefficient of variation. Table 6 shows that the standard deviation and coefficient of variation were in the ranges of 0.05-0.23 MPa and 2.1-9.9% (average of 6.2%), respectively. Thus, it can be concluded that the test results of the splitting tensile strength have relatively low dispersion, high repeatability, and high precision, but are associated with slightly more uncertainty than those of the compressive strength.
5. Conclusions
The combined effect of basalt fiber addition and RCA replacement on the workability and early-age compressive and splitting tensile strength has been investigated. Two lengths of basalt fibers were used, namely 20 and 43 mm. The concrete design strengths were set to 30, 45, and 60 MPa. The following conclusions can be drawn based on experimental findings:
- The replacement of 100% RCA in concrete did not affect the workability of mixes with 30 and 45 MPa design strength, but caused a 16% reduction for the 60-MPa counterpart. The addition of BF reduced the workability. Increased volume fractions and length of the fibers reduced the workability.
- The substitution of NA by 100% RCA led to a decrease in the concrete compressive strength. This strength reduction was more pronounced in concrete with a higher design strength. The incorporation of 20- and 43-mm basalt fibers in 100% RCA concrete increased the compressive strength of RCA-concrete by up to 37 and 39%, respectively. However, the strength was lesser than that of the control mixes. In fact, the mixes made with 20- and 43-mm basalt fiber could only retain up to 70 and 58% of the strength of the control mixes. Nevertheless, the 20-mm basalt fibers seemed to be more impactful when used in concrete with a lower design strength, while the 43-mm counterparts were more effective in concrete with higher design strengths.
- The splitting tensile strength decreased upon the replacement of NA by 100% RCA for concrete irrespective of the concrete design strength. However, the effect of RCA replacement was less detrimental on the splitting tensile strength than the compressive strength. Compared to the control mixes, the addition of 20- and 43-mm basalt fibers increased the splitting tensile strength by up to 117 and 147%, respectively, with a higher percent increase being noted for concrete with a lower design strength. Also, the 43-mm basalt fiber-reinforced concrete had a higher splitting tensile strength than that of 20-mm counterparts.
Table 7. Splitting tensile strength of BF-reinforced concrete
Mix Designation | Avg. ft (MPa) | Increase in ft compared to RCA concrete (%) | Achieved ft of BFRC as percent of NC (%) | f t /fcu (%) |
30R0BF0 | 1.7 | - | - | 5.0 |
30R100BF0 | 1.3 | - | - | 7.6 |
30R100BF0.5/43 | 1.9 | 45 | 114 | 8.6 |
30R100BF1/43 | 2.4 | 86 | 147 | 11.7 |
30R100BF0.5/20 | 1.8 | 36 | 107 | 7.5 |
30R100BF1/20 | 1.6 | 25 | 98 | 8.6 |
30R100BF1.5/20 | 1.9 | 42 | 112 | 8.4 |
30R100BF2/20 | 1.7 | 30 | 102 | 7.9 |
30R100BF3/20 | 1.9 | 48 | 117 | 9.6 |
45R0BF0 | 2.3 | - | - | 5.1 |
45R100BF0 | 1.5 | - | - | 6.2 |
45R100BF0.5/43 | 2.2 | 47 | 95 | 8.0 |
45R100BF1/43 | 3.1 | 109 | 135 | 11.2 |
45R100BF0.5/20 | 2.3 | 56 | 101 | 8.5 |
45R100BF1/20 | 1.9 | 30 | 84 | 7.0 |
45R100BF1.5/20 | 2.3 | 56 | 101 | 8.5 |
45R100BF2/20 | 2.4 | 60 | 104 | 8.7 |
45R100BF3/20 | 2.6 | 74 | 113 | 10.1 |
60R0BF0 | 2.5 | 4.7 | ||
60R100BF0 | 1.5 | - | - | 6.9 |
60R100BF0.5/43 | 2.2 | 45 | 89 | 8.0 |
60R100BF1/43 | 3.3 | 116 | 132 | 10.7 |
60R100BF0.5/20 | 1.7 | 13 | 69 | 7.0 |
60R100BF1/20 | 1.6 | 8 | 66 | 6.5 |
60R100BF1.5/20 | 2.4 | 58 | 97 | 8.2 |
60R100BF2/20 | 2.5 | 61 | 99 | 8.4 |
60R100BF3/20 | 2.9 | 89 | 115 | 10.6 |
Acknowledgements
The authors gratefully acknowledge the financial support provided by UAE University under grants number 31N398 and 31N324.
References
[1] M. Roser, H. Ritchie, and E. Ortiz-Ospina, “World Population Growth,” Our World Data, May 2013, Accessed: Dec. 11, 2020. [Online].Available: View Article
[2] J. Van Bavel, “The world population explosion: causes, backgrounds and projections for the future,” Facts Views Vis. ObGyn, vol. 5, no. 4, pp. 281–291, 2013.
[3] C. R. Gagg, “Cement and concrete as an engineering material: An historic appraisal and case study analysis,” Eng. Fail. Anal., vol. 40, pp. 114–140, 2014. View Article
[4] P. D. P. Kumar Mehta and P. D. Paulo J. M. Monteiro, Concrete: Microstructure, Properties, and Materials, Fourth Edition . McGraw-Hill Education: New York, Chicago, San Francisco, Athens, London, Madrid, Mexico City, Milan, New Delhi, Singapore, Sydney, Toronto, 2014.
[5] J. Newman and B. S. Choo (Eds), Advanced Concrete Technology, 1st Edition. Elsevier, 2003.
[6] V. Radonjanin, M. Malešev, S. Marinković, and A. E. S. Al Malty, “Green recycled aggregate concrete,” Constr. Build. Mater., vol. 47, pp. 1503–1511, 2013. View Article
[7] S. Marinković, V. Radonjanin, M. Malešev, and I. Ignjatović, “Comparative environmental assessment of natural and recycled aggregate concrete,” Waste Manag., vol. 30, no. 11, pp. 2255–2264, 2010. View Article
[8] N. Bandow, S. Gartiser, O. Ilvonen, and U. Schoknecht, “Evaluation of the impact of construction products on the environment by leaching of possibly hazardous substances,” Environ. Sci. Eur., vol. 30, no. 1, p. 14, 2018. View Article
[9] N. V. Bakaeva and M. Y. Klimenko, “Technique for Reduction of Environmental Pollution from Construction Wastes,” IOP Conf. Ser. Mater. Sci. Eng., vol. 262, no. 1, p. 012195, 2017. View Article
[10] A. Masood, T. Ahmad, M. Arif, and F. Mahdi, “Waste management strategies for concrete,” Environ. Eng. Policy, vol. 3, no. 1, pp. 15–18, 2001. View Article
[11] H. Guo, C. Shi, X. Guan, J. Zhu, Y. Ding, T. C. Ling, H. Zhang, and Y. Wang, “Durability of recycled aggregate concrete – A review,” Cem. Concr. Compos., vol. 89, pp. 251–259, 2018. View Article
[12] E. E. Anike, M. Saidani, E. Ganjian, M. Tyrer, and A. O. Olubanwo, “The potency of recycled aggregate in new concrete: a review,” Constr. Innov., vol. 19, no. 4, pp. 594–613, 2019,. View Article
[13] M. Chakradhara Rao, S. K. Bhattacharyya, and S. V. Barai, “Influence of field recycled coarse aggregate on properties of concrete,” Mater. Struct., vol. 44, no. 1, pp. 205–220, 2011. View Article
[14] S. C. Kou and C. S. Poon, “Enhancing the durability properties of concrete prepared with coarse recycled aggregate,” Constr. Build. Mater., vol. 35, pp. 69–76, 2012. View Article
[15] N. K. Bairagi, K. Ravande, and V. K. Pareek, “Behaviour of concrete with different proportions of natural and recycled aggregates,” Resour. Conserv. Recycl., vol. 9, no. 1, pp. 109–126, 1993. View Article
[16] M. Malešev, V. Radonjanin, and S. Marinković, “Recycled Concrete as Aggregate for Structural Concrete Production,” Sustainability, vol. 2, no. 5, Art. no. 5, 2010. View Article
[17] M. B. de Oliveira and E. Vazquez, “The influence of retained moisture in aggregates from recycling on the properties of new hardened concrete,” Waste Manag., vol. 16, no. 1, pp. 113–117, 1996. View Article
[18] S.-C. Kou and C.-S. Poon, “Long-term mechanical and durability properties of recycled aggregate concrete prepared with the incorporation of fly ash,” Cem. Concr. Compos., vol. 37, pp. 12–19, 2013. View Article
[19] M. Bravo, J. de Brito, J. Pontes, and L. Evangelista, “Durability performance of concrete with recycled aggregates from construction and demolition waste plants,” Constr. Build. Mater., vol. 77, pp. 357–369, 2015. View Article
[20] T. Vieira, A. Alves, J. de Brito, J. R. Correia, and R. V. Silva, “Durability-related performance of concrete containing fine recycled aggregates from crushed bricks and sanitary ware,” Mater. Des., vol. 90, pp. 767–776, 2016. View Article
[21] N. Kachouh, H. El-Hassan, and T. El-Maaddawy, “Effect of steel fibers on the performance of concrete made with recycled concrete aggregates and dune sand,” Constr. Build. Mater., vol. 213, pp. 348–359, 2019. View Article
[22] N. Kachouh, H. El-Hassan, and T. El Maaddawy “Influence of steel fibers on the flexural performance of concrete incorporating recycled concrete aggregates and dune sand,” J. Sust. Cem. Based Mater., pp. 1–28, 2020. View Article
[23] J. A. Carneiro, P. R. L. Lima, M. B. Leite, and R. D. Toledo Filho, “Compressive stress–strain behavior of steel fiber reinforced-recycled aggregate concrete,” Cem. Concr. Compos., vol. 46, pp. 65–72, 2014. View Article
[24] S. U. Balouch, J. P. Forth, and J.-L. Granju, “Surface corrosion of steel fibre reinforced concrete,” Cem. Concr. Res., vol. 40, no. 3, pp. 410–414, 2010. View Article
[25] Y. Wang, P. Hughes, H. Niu, and Y. Fan, “A new method to improve the properties of recycled aggregate concrete: Composite addition of basalt fiber and nano-silica,” J. Clean. Prod., vol. 236, p. 117602, 2019. View Article
[26] S.-E. Fang, H.-S. Hong, and P.-H. Zhang, “Mechanical Property Tests and Strength Formulas of Basalt Fiber Reinforced Recycled Aggregate Concrete,” Mater., vol. 11, no. 10, 2018. View Article
[27] J. F. Dong, Q. Y. Wang, Z. W. Guan, " Material properties of basalt fibre reinforced concrete made with recycled earthquake waste", Constr. Build. Mater., vol. 130, pp. 241-241, 2017. View Article
[28] ASTM C136, “Test Method for Sieve Analysis of Fine and Coarse Aggregates,” ASTM International. View Article
[29] ASTM C88, “Test Method for Soundness of Aggregates by Use of Sodium Sulfate or Magnesium Sulfate,” ASTM International. View Article
[30] ASTM C127, “Test Method for Relative Density (Specific Gravity) and Absorption of Coarse Aggregate,” ASTM International. View Article
[31] ASTM C29, “Test Method for Bulk Density (Unit Weight) and Voids in Aggregate,” ASTM International. View Article
[32] ASTM C131, “Test Method for Resistance to Degradation of Small-Size Coarse Aggregate by Abrasion and Impact in the Los Angeles Machine,” ASTM International. View Article
[33] ReforceTech, “MiniBarsTM Data Sheet.” (accessed Dec. 14, 2020). View Article
[34] ACI 211.1, “Standard Practice for Selecting Proportions for Normal, Heavyweight, and Mass Concrete (Reapproved 2009).” (accessed Jan. 23, 2020). View Article
[35] ASTM C143, “Test Method for Slump of Hydraulic-Cement Concrete,” ASTM International. View Article
[36] British Standards, “BS 1881-124:2015 - Testing concrete. Methods for analysis of hardened concrete.” (accessed Feb. 09, 2020). View Article
[37] ASTM C496, “Test Method for Splitting Tensile Strength of Cylindrical Concrete Specimens,” ASTM International. View Article
[38] Safiuddin, U. J. Alengaram, A. Salam, M. Z. Jumaat, F. F. Jaafar, and H. B. Saad, “Properties of high-workability concrete with recycled concrete aggregate,” Mater. Res., vol. 14, no. 2, pp. 248–255, 2011. View Article