Volume 5 - Year 2022- Pages 78-84
DOI: 10.11159/ijci.2022.011
Assessing the Effect of Coarse Aggregate Size on Self-Compacting Fibre Reinforced Concrete Mix
Abdulkarim Mimoun1 and Sivakumar Kulasegaram2
1,2School of Engineering
Cardiff University
Newport Road, Cardiff CF24 3AA, United Kingdom
MimounAA@cardiff.ac.uk; KulasegaramS@cardiff.ac.uk
Abstract - Steel fibres increase inhomogeneity and alter rheological and hardened characteristics of self-compacting concrete. To investigate the rheological behaviour and hardened characteristics of self-compacting concrete (SCC) and self-compacting steel fibre reinforced concrete (SCSFRC), a wide range of normal strength self-compacting concrete mixes containing steel fibres and coarse aggregates (of size 10 mm, 20 mm) with target cube compressive strengths between 30 to 70 MPa were prepared in the laboratory. The plastic viscosity of these concrete mixes were estimated to be in the range between 20–50 Pa s , and the slump flow time t500 of each mix was recorded to ensure that the flow and passing ability for workability of the mixes satisfy the recommended British and European standards. This work mainly focuses on the properties of fibre reinforced self-compacting concrete containing 0.5% and 1% (by volume fraction) steel fibres and the effect of coarse aggregates on their rheological behaviour and flow characteristics. The effect of steel fibre content on the strength of hardened concrete is also investigated. In addition, comparison of flow behaviour between SCC and SCSFRC is also presented.
Keywords: Steel fibre, Self-compacting steel fibre reinforced concrete, Mix design, Plastic-viscosity.
© Copyright 2022 Authors - This is an Open Access article published under the Creative Commons Attribution License terms. Unrestricted use, distribution, and reproduction in any medium are permitted, provided the original work is properly cited.
Date Received: 2022-06-13
Date Accepted: 2022-06-20
Date Published: 2022-07-12
1. Introduction
Self-compacting concrete (SCC) flows under its own weight, without vibration effort, bleeding or segregation, and it has ability to fill formworks evenly [1]. Hence, it is a suitable structural material for frames with highly congested reinforcement or complex geometries [2][3]. To attain satisfactory SCC mix, the concrete mix must be stable and to avoid segregation it should possess favourable rheological properties [4]. Therefore, viscosity-modifying agent (e.g. superplasticizer), additional cementitious materials (ACMs), natural pozzolans, and fly ash etc. are necessary elements to maintain suitable water to binder ratio for decreasing the production cost and for maintaining optimal viscosity of SCC [5]. The properties of SCC mix with steel fibres, both in its fresh and hardened states, substantially depend on the composition and characteristics of its components. The prediction of SCC flow including its passing behaviour is very challenging particularly in the presence of congested reinforcing steel bars and in formworks of complex shapes. Nevertheless, a comprehensive understanding of rheological behaviour of SCC flow and its passing ability is crucial to achieve a high-quality Self-compacting steel fibre reinforced concrete (SCSFRC). The most pragmatic way to achieve such an understanding is by performing laboratory experiments, which will enable one to fully understand the flow, passing and filling ability behaviour of SCSFRC and to establish its characteristic strength.
The attractive properties of self-compacting concrete (SCC) are further enhanced by fibres which bridge cracks thus delaying their spread and hence improve the tensile strength, fracture toughness, and flexural strength of hardened SCC. Consequently, the utilization of fibres may expand the potential scope of application of SCC. SCSFRC has attracted wider interest because of the advantages of self-compacting behaviour in construction methods, and its hardened characteristics include being more ductile, with higher residual tensile strength compared to vibrated concrete [6]. The characteristics of steel fibre-reinforced compounds are largely established by the fibre aspect ratio, the fibre content, and the characteristic of fibre distribution within the matrix itself. The effectiveness of fibres on hardened properties of SCSFRC will depend on how the fibres are distributed within the structures cast. For this reason, it is important to investigate the distribution of steel fibre to ensure that mix design can yield evenly and favourably distributed fibres for obtaining optimum structural performance from SCSFRC.
The main aim of this paper is to analyse a wide range of normal strength SCSFRC mixes with coarse aggregates (of size 10 and 20 mm) and target cube compressive strength between 30 to 70 MPa. The target plastic viscosities of these mixes were estimated to be between 20 to 50 Pa s. The traditional slump cone, and J-ring tests were conducted to ensure that each mix met the flow, filling and passing ability criteria and, there was no segregation (BS EN 206-9: 2010) [7]. First step in this investigation is to assess how the estimated plastic viscosity (PV) of the self-compacting concrete mixes with and without steel fibres relates to fresh and hardened states flow/mechanical properties of the developed mixes. Secondly, the effect of steel fibre volume fractions (i.e., 0.5%, 1.0%) and the size of coarse aggregates (i.e., 10 mm and 20 mm) on the properties of SCSFRC mixes were explored.
2. Development of the self-compacting concrete mixes with and without steel fibres
An extensive laboratory study was conducted to develop various low and moderate strength SCC mix containing steel fibres (of 30 mm length and 0.55 mm diameter) with nominal 28-day cube compressive strengths of 30, 40, 50, 60 and 70 MPa. In these mixes coarse aggregates with 10 and 20 mm sizes were used. All mixes were assessed for flow quality in their fresh state utilizing slump cone and J-ring tests. This procedure ensured that each mix was tested to satisfy the flowability, passing ability criteria and showed no visible signs of segregation as recommended by (BSI, 2010; EFNARC, 2005) [7] [8]. The development of these mixes followed a mix design procedure, which is based on the plastic viscosity and compressive strength of the SCSFRC mixes, in accordance with the rational mix design method proposed by Abo Dhaheer et al. (2016a, 2016b) [9] [10]. This mix design procedure rationalised and simplified the method previously recommended by Karihaloo and Ghanbari (2012) [11] and Deeb and Karihaloo (2013) [12].
The amounts and specification of the components used in the SCC mixes are shown in Table 1 and Table 2. In this article test mixes are denoted by letter ‘C’ followed by their corresponding target compressive strength measured in MPa. Portland limestone cement (CEM II/A-L/32.5R) conforming to [13] with a specific gravity of 2.95 and Ground granulated blast-furnace slag (GGBS) with a specific gravity of 2.40 were used as the main cement and cement replacement material respectively. A new generation of polycarboxylic ether-based superplasticiser (SP) with specific gravity of 1.07 was used in all the test mixes. Crushed limestone coarse aggregate with maximum particle size of 20 mm and a specific gravity of 2.80 was used, while the fine aggregate (FA) was river sand (of less than 2 mm in size) having a specific gravity of 2.65. Limestone powder (LP) as a filler with maximum particle size of 125 μm (specific gravity 2.40) was used. A part of the river sand was substituted by an equal amount of the coarser fraction of LP in the size range 125 μm – 2 mm.
Table 1: Mix proportions of SCSFRC mixes (kg/m3)
Mix |
Cement |
GGBS |
Water |
SP |
LP (size <125 μm) |
SF (0.5 % vol. fract.) |
FA (< 2 mm)1 |
CA (size < 20 mm) |
|
FA** |
FA*** |
||||||||
C30 |
244 |
81 |
205 |
2.7 |
158 |
40 |
218 |
550 |
827 |
C50 |
275 |
92 |
195 |
2.8 |
207 |
38 |
189 |
600 |
842 |
C70 |
338 |
113 |
180 |
3.8 |
164 |
38 |
216 |
500 |
819 |
1Note: A part of the fine aggregate is the coarser fraction of the limestone powder FA**125 μm–2 mm, whereas FA*** refers to natural river sand < 2 mm.
Table 2:Further details of SCSFRC mix proportions
Mix |
water-to- cementitious material ratio (w/cm) |
Superplasticizer -to- cementitious material ratio (SP/cm) |
C30 |
0.63 |
0.65 |
C50 |
0.52 |
0.76 |
C70 |
0.40 |
084 |
The plastic viscosity of each mix was estimated using micro-mechanical theory based analytical procedure described in Ghanbari and Karihaloo (2009) [14] and the plastic viscosity of the homogeneous paste was obtained from (Sun et al., 2006) [15] and [9][10][16][17]. Additional details of the developed SCSFRC test mixes are presented in Table 3. The results obtained from slump flow and J-ring tests are detailed in Table 4 which confirms that all the SCSFRC mixes with 0.5% volume fraction of steel fibres satisfy the fresh state flow criteria [7] [8].
Table 3: Further details of test SCSFRC mixes
Mix |
Compressive strength at 28 days (MPa) |
Estimated Plastic viscosity (Pa s) |
Paste vol. fraction |
Solid vol. fraction |
Paste/Solid (By vol.) |
C30 |
41.77 |
20 |
0.39 |
0.61 |
0.64 |
C50 |
50.20 |
35 |
0.41 |
0.59 |
0.71 |
C70 |
78.43 |
45 |
0.44 |
0.56 |
0.80 |
Table 4: Flow test results for SCSFRC mixes
Mix |
Slump flow |
J-ring flow test (12 Bars) |
DF - DJ (mm)* |
|||
Spread DF (mm) |
Time 500 mm (sec) |
Spread DJ (mm) |
Time 500 mm (sec) |
PJ (mm) |
||
C30 |
632.5 |
2.16 |
630 |
2.50 |
9.50 |
2.5 |
C50 |
635 |
2.47 |
625 |
3.47 |
7.75 |
10 |
C70 |
750 |
2.67 |
745 |
3.50 |
7 |
5 |
* Difference between Slump flow and J-ring flow test
3. Estimation of mix plastic viscosity
The flow of SCC with or without steel fibre is best illustrated by a Bingham constitutive model. This model comprises two material properties, specifically the yield stress (τy) and the plastic viscosity (PV). It is recognised however that the yield stress of SCC mixtures is very low (around 200 Pa) in comparison with normal concretes (thousands of Pascal) and remains approximately constant for a large range of plastic viscosities [16]. The increase in plastic viscosity of the liquid phase (cement paste), as a consequence of adding solid components such as aggregates and fibres, can be estimated by means of a two-stage micro-mechanical model. It takes into account the shape and the volume fraction of the solid suspension particles [17].
The viscosity of a homogenous viscous fluid for example the paste of cement (liquid phase) can be measured accurately, which cannot be said about fluids of non-homogeneous viscous (solid phase) such as SCC and SCSFRC. Nevertheless, the plastic viscosity of SCC with or without steel fibres can be obtained by a micromechanical procedure from the knowledge of the plastic viscosity of cement paste alone or of the viscosity modifying admixture (VMA) and/or cement paste with SP. This process has been proven to predict the plastic viscosity of SCC mixes with and without fibres that agree very well with measured values [14].
This model involves treating SCSFRC as a two-phase suspension in which the solid phase is suspended in a viscous liquid phase. The plastic viscosity of the liquid phase can be measured accurately using a rheometer. The increase in the plastic viscosity of the paste resulted by the addition of the solid phase can be predicted in phases from the two-phase suspension model.
The plastic viscosity of SCSFRC increases due to the inclusion of fibres, to a degree based on the structure of the reference mix [18]. The viscosity of SCC mixtures can be estimated using a micromechanical method, depending on the measured viscosity of the mix ratios and the cement paste. It is reported in [16] that the 30 mm long, 0.55 mm diameter SF with crimped ends considerably increased the viscosity of SCC with fibre mixes.
It is worth mentioning that the presence of steel fibres significantly increases the plastic viscosity of SCSFRC with various compressive strengths. Accordingly, the plastic viscosity of the viscous concrete comprising liquid and solid phases is additionally increased if SF are added to it. In the present work, the amount of volume fraction of steel fibres is typically small (i.e. less than 1%), so that the dilute approximation for fibre content is still applicable during the estimation of plastic viscosity based on micro-mechanical theory [14].
4. The effect of steel fibre content and the size of coarse aggregate on SCC and SCSFRC mixes
The steel fibre content (i.e., its volume fraction) and the maximum size of coarse aggregates will have significant effect on self-compacting concrete mix, as well as on the distribution and orientation of steel fibres in terms of its flow, passing, and filling ability. This will in turn influence both fresh and hardened properties of SCSFRC. The characteristics of steel fibre and maximum size of coarse aggregate (i.e., 20 mm in the present work) will largely govern the SCSFRC flow properties and homogeneity of the mix. With increasing steel fibre content and size of coarse aggregate, the strength of SCSFRC increases while workability decreases, which may possibly fail to meet the EFNARC standard [8].
Table 5 presents constituents and proportions of various SCSFRC concrete mixes containing 1.0 % (by volume) of steel fibres with target compressive strength 30 MPa.
Table 5: SCSFRC mixes with varying CA size (kg/m3)
Mix |
C30 (1) |
C30 (2) |
C30 (3) |
C30 (4) |
|
Cement |
247 |
247 |
247 |
328 |
|
GGBS |
82 |
82 |
82 |
-- |
|
Micro-silica |
-- |
-- |
-- |
58 |
|
Water |
207 |
207 |
207 |
243 |
|
SP |
2.8 |
2.8 |
2.8 |
4.75 |
|
LP (size <125 μm) |
278 |
278 |
278 |
366 |
|
SF (1.0% vol. fract.) |
76 |
76 |
76 |
103 |
|
FA(< 2 mm)1 |
-- |
-- |
-- |
-- |
|
FA** |
205 |
205 |
205 |
446 |
|
FA*** |
500 |
500 |
500 |
700 |
|
CA (size < 20 mm) |
364.5 (50%) 10mm |
546.75 (75%) 10mm |
546.75 (75%) 10mm |
546.75 (75%) 10mm |
|
364.5 (50%) 20 mm |
182.25 (25%) 20 mm |
182.25 (25%) 20 mm |
182.25 (25%) 20 mm |
||
Water-to- cementitious material ratio (w/cm) |
0.63 |
0.63 |
0.63 |
0.63 |
|
Superplasticizer -to- cementitious material ratio (SP/cm) |
0.86 |
0.86 |
0.86 |
1.23 |
1Note: A part of the fine aggregate is the coarser fraction of the limestone powder) FA**125 μm–2 mm, whereas FA*** refers to natural river sand < 2 mm), h Coarse aggregate < 20 mm and < 10 mm.
The results obtained during the fresh state tests of the mixes listed in Table 5 are given in Table 6. The final spread diameter values are within the range of 600 mm to 750 mm for flow and passing ability tests in case of all the SCSFRC mixes in Table 5. However, it can be noted that in the case of mix (1), the PJ value (i.e., blocking step height) is rather high indicating that there is a significant blocking and segregation of steel fibre and coarse aggregates. In addition, it can be also noted that for mix (2) and (3) the PJ value is greater than 10mm which again confirms that the flow is slightly blocked during the J-ring test. This fact is further established by L-box tests. Figure 1 illustrates the L-box test results for all the mixes in Table 5. As noted with J-ring tests, the L-box tests reveal that the flow of mix (1), (2) and (3) are subjected to blockage and segregation of fibres and coarse aggregates. As mix (4) does not contain any coarse aggregate, there is no visible blockage in the case of mix (4). Further, it can be noted that the value of PJ in case of mix (4) is 6.25mm which is less than the maximum recommended value (i.e., 10 mm) for self-compacting concrete.
Table 6: Flow test results for SCSFRC mixes listed in Table 5
Mix |
Slump flow |
J-ring flow test (12 Bars) |
DF - DJ (mm)* |
L-box test |
|||||
Spread DF (mm) |
Time 500mm (sec) |
Spread DJ (mm) |
Time 500mm (sec) |
PJ (mm) |
t200 (sec) |
t400 (sec) |
H2/H1 |
||
C30 (1) |
630 |
1.97 |
615 |
3.50 |
24.5 |
15 |
1.17 |
2.33 |
0.88 |
C30 (2) |
610 |
2.37 |
605 |
3.14 |
17 |
5 |
1.00 |
2.77 |
0.90 |
C30 (3) |
605 |
1.99 |
600 |
3.05 |
12 |
5 |
1.01 |
2.50 |
0.89 |
C30 (4) |
620 |
2.34 |
610 |
3.10 |
6.25 |
10 |
0.63 |
1.70 |
0.94 |
* Difference between Slump flow and J-ring flow test
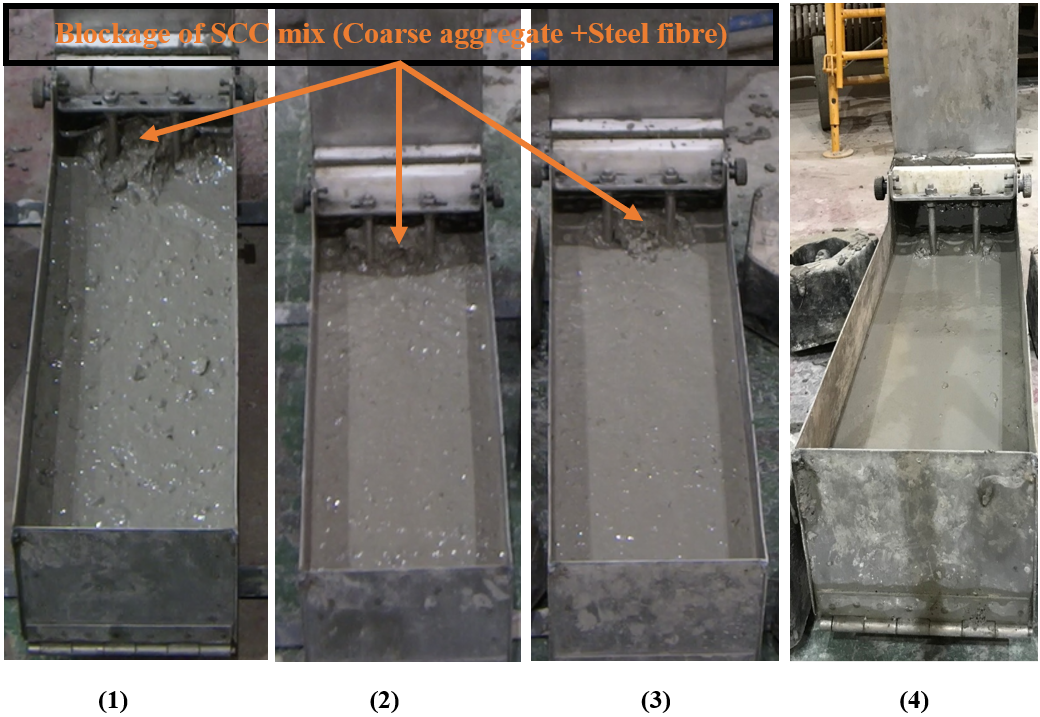
To further investigate the properties of SCC and SCSFRC mix, SCC and SCSFRC mixes with target compressive strength of 40 MPa and 60 MPa were considered following the analysis of 30MPa mixes listed above. For both SCC and SCSFRC mixes (mixes 40 MPa and 60 MPa) in Table 7, the water-to-binder ratio was reduced (to 0.57 and 0.47 respectively), while the superplasticiser to cementitious materials ratio (SP/cm) was increased to ensure good workability. Target compressive strength is slightly increased for SCSFRC mixes with increased plastic viscosity.
Table 7 presents constituents and mix proportions of 40 MPa and 60 MPa mixes with and without steel fibres. As can be noted from the Table 7, mixes with fibres contain 0.5% (by volume) steel fibres and all the mixes contain 10 mm, and 20 mm coarse aggregates of equal amounts (i.e., 50% each of total coarse aggregate). Table 8 details the results obtained during the fresh state slump flow and J-ring tests carried out with the mixes listed in Table 7. The flow spread diameter values are within the range between 600 mm and 650 mm thus confirming satisfactory flowability of SCSFRC mixes. This observation is consistent in the case of both 12 bar and 16 bar J-ring tests according to BS EN 206-9:2010 [7]. However, it can be noted from Figure 2 that the PJ value for J-ring test with 16 bar is greater than 10 mm. This indicates that the there is a possibility of blockage and segregation during the flow of SCSFRC. Figure 2 clearly illustrates the flow blockage and the segregation of coarse aggregates. Therefore, it is suggested that by decreasing the amount of coarse aggregate, the passing ability can be further improved as in the case of 30 MPa mixes presented above. However, it is important to ensure that the reduction is coarse aggregate does not affect the predicted compressive strength of hardened concrete.
L-box test can be performed with 2 or 3 steel re-bars for assessing passing and filling ability of SCSFRC. The L-box tests reveal that the blocking of steel fibre and coarse aggregates (size from 10 mm to 20 mm) was observed with 3 bars as can be seen from Figure 3, while with 2 re-bars the mixtures showed no signs of blocking or segregation. At the same time, during L-box test with 3 re-bars, the mixtures without steel fibres (and with equal amount of 10 mm and 20 mm coarse aggregates) no signs of blocking or segregation was observed as shown in Figure 3. This means that the large aggregate could take the space of steel fibre and cementitious paste due to blocking. Furthermore, the cementitious paste should be of increased mix with steel fibre in order to play its role in aspects of distribution and orientation, especially in congestion reinforcement.
Table 7: Mix proportions for SCC and SCSFRC mixes (kg/m3)
Mix |
C40 |
C40 (0.5% SF) |
C60 |
C60 (0.5% SF) |
Cement |
288 |
270 |
315 |
303 |
GGBS |
95 |
90 |
105 |
101 |
Water |
219 |
205 |
198 |
190 |
SP |
2.5 |
3 |
2.6 |
3.5 |
LP (size <125 μm) |
130 |
143 |
140 |
129 |
SF (0.5% vol. fract.) |
-- |
40 |
-- |
39 |
FA(< 2 mm)1 |
-- |
-- |
-- |
-- |
FA** |
200 |
240 |
198 |
210 |
FA*** |
544 |
500 |
500 |
500 |
CA (size < 20 mm |
770 |
839 |
862 |
887 |
water-to- cementitious material ratio (w/cm |
0.57 |
0.57 |
0.47 |
0.47 |
Superplasticizer -to- cementitious material ratio (SP/cm) |
0.78 |
0.83 |
0.62 |
0.86 |
1Note: A part of the fine aggregate is the coarser fraction of the limestone powder FA**125 μm–2 mm, whereas FA*** refers to natural river sand < 2 mm.
Table 8: Flow test results for SCC and SCSFRC mixes
Mix |
Slump flow |
J-ring flow test |
DF- DJ (mm)* |
L-box test |
|||||
Spread (mm) |
Time 500mm (sec) |
Spread (mm) |
Time 500mm (sec) |
PJ (mm) |
t200 (sec) |
t400 (sec) |
H2/H1 |
||
C40 |
602 |
1.20 |
600 |
2.02 |
9.5 |
2 |
0.53 |
1.27 |
0.93 |
C40 (SF) |
635 |
2.27 |
615 |
2.54 |
7.7 |
20 |
0.93 |
1.90 |
0.97 |
C60 |
625 |
1.41 |
620 |
1.73 |
7.7 |
5 |
0.77 |
1.60 |
0.94 |
C60 (SF) |
645 |
2.50 |
625 |
3.37 |
7 |
20 |
1.15 |
2.55 |
0.91 |
* Difference between Slump flow and J-ring flow test
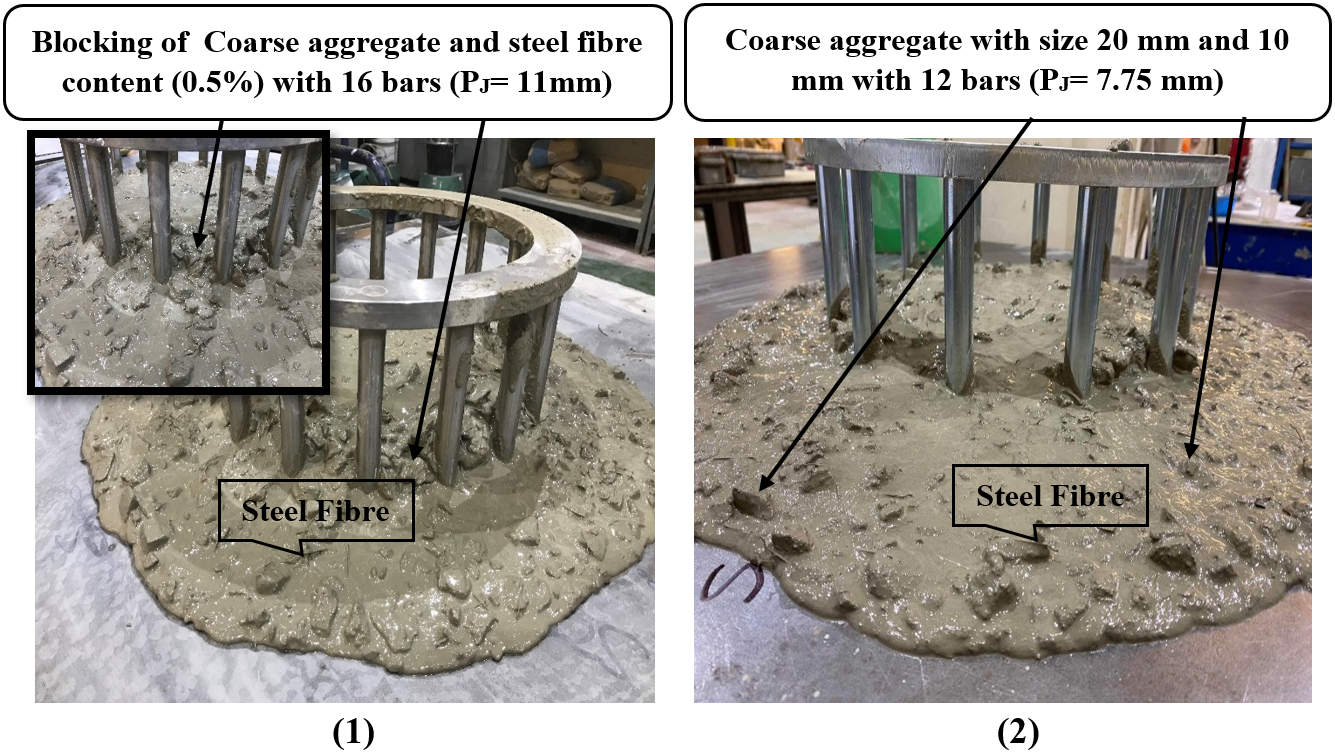
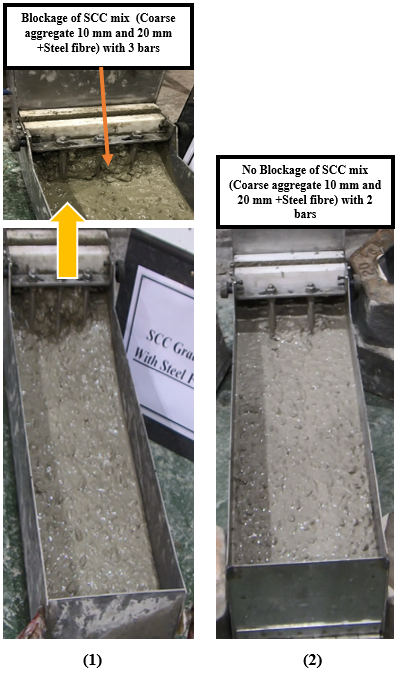
5. Conclusion
This paper focused on developing self-compacting steel fibre reinforced concrete mixes and characterising their flow and mechanical properties. To understand the effect of steel fibres and coarse aggregates on SCC properties, mixes with target compressive strengths between 30 to 70 MPa were prepared and tested in the laboratory. The experimental results reveal that with the increase in the amount of 20mm aggregate, the blocking step height increases during the J-ring tests especially in the presence of steel fibres with higher volume fraction (i.e., 1 %). This observation clearly indicates that the coarse aggregate (e.g., 20mm and 10mm) composition should be carefully optimised to obtain favourable self-compacting and mechanical properties of SCSFRC.
References
[1] H. Okamura and M. Ouchi, “Self-Compacting Concrete,” J. Adv. Concr. Technol., vol. 1, no. 1, pp. 5–15, 2003, doi: 10.3151/jact.1.5. View Article
[2] F. Aslani, “Effects of specimen size and shape on compressive and tensile strengths of selfcompacting concrete with or without fibres,” Mag. Concr. Res., vol. 65, no. 15, pp. 914–929, 2013, doi: 10.1680/macr.13.00016. View Article
[3] F. Aslani and J. Kelin, “Assessment and development of high-performance fibre-reinforced lightweight self-compacting concrete including recycled crumb rubber aggregates exposed to elevated temperatures,” J. Clean. Prod., vol. 200, pp. 1009–1025, 2018, doi: 10.1016/j.jclepro.2018.07.323. View Article
[4] K. H. Khayat, “Workability , Testing , and Performance of Self-Consolidating,” no. May 1999, 2015.
[5] M. Sahmaran and I. O. Yaman, “Hybrid fiber reinforced self-compacting concrete with a high-volume coarse fly ash,” vol. 21, pp. 150–156, 2007, doi: 10.1016/j.conbuildmat.2005.06.032. View Article
[6] X. Ding, C. Li, B. Han, Y. Lu, and S. Zhao, “Effects of different deformed steel-fibers on preparation and fundamental properties of self-compacting SFRC,” Constr. Build. Mater., vol. 168, pp. 471–481, 2018, doi: 10.1016/j.conbuildmat.2018.02.162. View Article
[7] EN BS 206-9, “Additional Rules for Self- compacting Concrete (SCC).,” BSI Stand. Publ., 2010.
[8] “The European Guidelines for Self-Compacting Concrete Specification, Production and Use "The European Guidelines for Self Compacting Concrete",” 2005. Accessed: May 02, 2019. [Online]. Available: www.efnarc.org.
[9] W. S. et al. Abo Dhaheer, M. S. Al-Rubaye, M. M. Alyhya, “Proportioning of self–compacting concrete mixes based on target plastic viscosity and compressive strength: Part I - mix design procedure,” J. Sustain. Cem. Mater., vol. 5, no. 4, pp. 199–216, 2016, doi: 10.1080/21650373.2015.1039625. View Article
[10] M. S. Abo Dhaheer, M. M. Al-Rubaye, W. S. Alyhya, B. L. Karihaloo, and S. Kulasegaram, “Proportioning of self-compacting concrete mixes based on target plastic viscosity and compressive strength: Part II - experimental validation,” J. Sustain. Cem. Mater., vol. 5, no. 4, pp. 217–232, 2016, doi: 10.1080/21650373.2015.1036952. View Article
[11] B. L. Karihaloo and A. Ghanbari, “Mix proportioning of selfcompacting high-and ultrahigh-performance concretes with and without steel fibres,” Mag. Concr. Res., vol. 64, no. 12, pp. 1089–1100, 2012, doi: 10.1680/macr.11.00190. View Article
[12] R. Deeb and B. L. Karihaloo, “Mix proportioning of self-compacting normal and high-strength concretes,” Mag. Concr. Res., vol. 65, no. 9, pp. 546–556, 2013, doi: 10.1680/macr.12.00164. View Article
[13] BS EN 197-1, “Cemet : Composition, specifications and conformity criteria for common cements,” BSI, 2011.
[14] A. Ghanbari and B. L. Karihaloo, “Prediction of the plastic viscosity of self-compacting steel fibre reinforced concrete,” Cem. Concr. Res., vol. 39, no. 12, pp. 1209–1216, 2009, doi: 10.1016/j.cemconres.2009.08.018. View Article
[15] Z. Sun, T. Voigt, and S. P. Shah, “Rheometric and ultrasonic investigations of viscoelastic properties of fresh Portland cement pastes,” Cem. Concr. Res., vol. 36, no. 2, pp. 278–287, 2006, doi: 10.1016/j.cemconres.2005.08.007. View Article
[16] R. Deeb, A. Ghanbari, and B. L. Karihaloo, “Development of self-compacting high and ultra high performance concretes with and without steel fibres,” Cem. Concr. Compos., vol. 34, no. 2, pp. 185–190, 2012, doi: 10.1016/j.cemconcomp.2011.11.001. View Article
[17] Á. de la Rosa, E. Poveda, G. Ruiz, and H. Cifuentes, “Proportioning of self-compacting steel-fiber reinforced concrete mixes based on target plastic viscosity and compressive strength: Mix-design procedure & experimental validation,” Constr. Build. Mater., vol. 189file://, pp. 409–419, 2018, doi: 10.1016/j.conbuildmat.2018.09.006. View Article
[18] S. Grunewald, Performance-based design of self-compacting fibre reinforced concrete. 2004.