Volume 6 - Year 2023- Pages 41-50
DOI: 10.11159/ijci.2023.006
Methods of Asphalt Petrology: Determination of Permeability
M.Sc. Leandro Harries1, M.Sc. Maximilian Schütz11, M.Sc. David Kempf1,Prof. Dr.-Ing. Jia Liu1
1Technical University of Darmstadt, Institute of Transportation Infrastructure Engineering
Otto-Berndt-Straße 2, Darmstadt, Hessen, Germany 64287
lharries@vwb.tu-darmstadt.de; mschuetz@vwb.tu-darmstadt.de; dkempf@vwb.tu-darmstadt.de; jliu@vwb.tu-darmstadt.de
Abstract - The benefits and properties of porous asphalt (PA) pavements were initially summarised based on a literature review. Further, the influence of modifications using different combinations of carbon and cellulose fibre was shown by laboratory tests carried out by the Institute of Transportation Infrastructure Engineering of Technical University of Darmstadt.
The main focus of this paper is to characterise the permeability of porous asphalt by using asphalt petrology, a geology-based method adapted for analysing the internal structure of asphalt samples.
This method introduces new parameters, such as the void surface area subdivided into classes. These parameters allow further attributes of the asphalt to be inferred, as was shown for vertical permeability in this paper. To this end, a function was successfully developed and applied to predict the permeability of asphalt specimens.
© Copyright 2023 Authors - This is an Open Access article published under the Creative Commons Attribution License terms. Unrestricted use, distribution, and reproduction in any medium are permitted, provided the original work is properly cited.
Date Received: 2023-06-04
Date Revised: 2023-07-09
Date Accepted: 2023-07-13
Date Published: 2023-08-08
1. Introduction
1. 1. Motivation - and research goals
The ability of surfaces to drain water is increasingly relevant due to growing urban areas with sealed surfaces at the expense of permeable natural surfaces. This has, in turn, led to increased flooding problems causing the temporary loss of urban infrastructure and non-point pollution effects [1, 2]. Furthermore, the sizable share of impermeable surfaces alters the natural water cycle in urban areas [3].
PA drains surface water through its pores. The pores in PA are higher in volume and more interconnected compared to conventional asphalt [4]. Allowing rainwater to infiltrate the pavement surface can reduce the impact of diffuse pollution by filtering it through individual permeable layers [1] and gradually returning it to the natural water cycle via the groundwater [5].
The permeability of PA is sufficient to help manage even intense rainstorm events [6, 7], preventing infrastructure from becoming hazardous or unusable. Another benefit of PA is improved traffic safety due to reduced surface water and higher skid resistance versus conventional sealed asphalt [5].
In terms of vision, PA reduces splash and spray of rain water by 90 % and 95 %, respectively, significantly improving visibility [8]. Also, the porous asphalt enhances traffic safety, as demonstrated by King et al. [9] at a PA friction course in Louisiana, where traffic fatalities and accidents were reduced by 100 % and 76 %, respectively. In San Antonio, Texas, the number of traffic accidents during rain events was reduced by 51 % by using porous asphalt [10]. PA offers further benefits in warm and wet climates or seasons. The water within the pores evaporates with increasing ambient temperature and cools the surroundings, reducing the Urban Heat Island Effect (UHIE) [11].
The UHIE describes increased Temperatures in urban areas compared to the surrounding suburban and rural areas due to the thermophysical properties of the materials used in the “built environment” [12, 13, 14].They have a higher degree of absorption of solar radiation and a higher thermal storage capacity. This increase in stored thermal energy leads to a higher surface temperature and stronger thermal radiation of the materials leading to higher ambient temperatures. [12] The temperature rise has adverse effects on the materials themselves [15, 16] as well as negative ecological [17] and health effects for people living within the UHIE-affected area [18, 19]
The Institute of Transportation Infrastructure Engineering (ITIE) of the Technical University of Darmstadt has conducted research on the characterisation of the vertical permeability of porous asphalt by asphalt petrology methods in lieu of the more laborious methods currently in use.
Asphalt petrology offers many insights into the inner structure of asphalt, such as the aggregate’s contact angle, distribution and orientation. It can also provide an accurate estimation of asphalt’s void content-related characteristics, such as vertical and horizontal distribution of voids, which the more common immersion weighing method fails to provide. Asphalt petrology can also indicate the presence of a weak layer bond caused by large accumulations of air voids [20].
1. 2. Modification of PA
PA has a critical drawback: raveling – a loss of surface material over time due to PA’s reduced durability compared to densely graded asphalt [21]. To mitigate this, PA can be modified with fibres and other additives.
Different materials such as cellulose, polypropylene, polyester, glass, mineral, and carbon fibre [22, 23, 24] can be used to improve asphalt performance.
Cellulose fibres have a high surface area, allowing them to bind more bitumen [25] and prevent draining down [5].
This increased bitumen retention was assumed to reduce air void content [26], and indeed Afonso et al. [27] found that the rutting resistance was improved due to the high binder absorption. However, raveling resistance was not improved. In fact, cellulose fibres have negatively affected particle loss in Cantabro tests.
Carbon fibre has been investigated by several different authors [28, 29] thanks to its several interesting properties, such as a high tensile modulus, high tensile strength, high heat conductivity, high chemical stability, a low thermal expansion coefficient, high electric conductivity, and high thermal resistance [16]. The addition of carbon fibre has been shown to reduce asphalt’s air void content while improving its stability and deformation resistance [30, 31].
However, Gupta et al. [5] studied the impact of fibre on the permeability of PA and found a slight negative effect. Deposition of sediments on the pavement surface can cause clogging, which also reduces permeability and noise absorption ability of the PA mixtures [10, 21]. This is because a certain degree of permeability is required for the proper functioning of PA. Water permeable asphalts (WPA) help ensure this by allowing for higher permeability at lower mechanical resilience vis a vis other PAs [32].
1. 3. Methods for determining permeability
Several methods are available to determine the permeability of PA. Falling and constant head permeability measuring procedures are used extensively. Both tests employ a standpipe attached to the specimen to be examined with a water column placed in the standpipe on the test specimen.
Falling head permeability-based tests as described in ASTM PS 129-01 [33] constitute one key method used to determine permeability. They measure the head loss of the standpipe fitted to the specimen in a given timeframe and have been used in numerous studies, such as from Mohammed et al. [34] and Vivar and Haddock [35].
Variations of the falling head test exist, such as those conducted under a vacuum with a fully saturated specimen and a flexible wall [36]. The falling head test is most suitable for less permeable asphalt mixtures [37].
Cooley [37] recommends constant-head tests to be applied to more permeable asphalts intended to transmit water. Constant head tests use Darcy’s Law to determine a coefficient of permeability. While keeping the head constant, the flow of the water being drained is measured and then used to calculate the coefficient [38]:
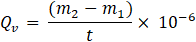
Qv = vertical water flow
m1 = Mass of the empty water container
m2 = mass of the water container after the test
t = test duration
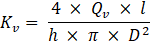
Kv = vertical permeability
l = width of the test specimen
h = height of water head
D = diameter of the test specimen
A more accurate assessment of the actual permeability of the PA in situ field tests should be conducted. Cooley and Brown [39] provided an example of a field test. This is especially important since field measurements do not exactly match lab measurements.
In addition to these direct attempts at measuring permeability, analytical models based on different characteristics have also been proposed. Alomari et al. [40] used the Kozeny-Carman-equation to calculate the permeability of hot mix asphalt (HMA) based on the air void (AV) content. The AV content, the tortuosity of flow paths and a surface area parameter were necessary for this computation. Using the same equation, Masad et al. [41] calculated the permeability using the AV content and the aggregate specific surface area. Alvarez et al. [36] took into account the influence of binder content on permeability by including the diameter of bitumen-covered aggregate. Based on the Masad et al. [41] study, AV content and average particle size appear to be the most important factors.
Alvarez et al. [36] found that crumb rubber-modified asphalt mixtures need more AV to attain a similar level of permeability as mixtures without this modification. For fibre conflicting findings exist. Afonso et al. [27] reported an improved permeability after applying cellulose fibre, while Lyons et al. [26] found a reduced permeability.
Król et al. [42] compared X-ray computed tomography (CT) methods to falling head laboratory methods to understand the relationship between air void content and structure from which permeability can be predicted. They concluded that CT could determine the permeability of asphalt based on the internal microstructure of porous asphalt.
This CT imaging technique was also used in an asphalt petrological study by Middendorf et al. [43], which stated that, even though CT imaging can assess the internal structure and permeability of asphalt, it is time intensive and requires costly equipment as well as specially trained personnel. Therefore, the CT method is best suited to limited and highly specialised cases.
1. 4. Asphalt Petrology
Asphalt petrology is a geology-based method for analysing the internal structure of asphalt samples. Initial experiments were carried out by the Danish Road Institute in the 1990s [44]. However, technical limitations at that time precluded the investigation of large-scale sections. To support advances in asphalt petrology, the Institute of Transportation Infrastructure Engineering at the Technical University of Darmstadt has been further developing micro-section analysis of asphalt at a teaching laboratory since 2017.
For image-analytical evaluation, the sample to be examined is first cut to the desired dimension using an automated precision saw. A slow and continuous feed of water is needed to remove the saw slurry and cool the specimen. This prevents scratching and smearing of the bitumen. The cut specimen is then placed in a mould made of foil, which lies as tightly as possible against the unprepared surfaces. In the next step, the specimen is placed in a vacuum chamber with pigmented epoxy resin that can penetrate even the smallest cavities under negative pressure. After curing, the resin supernatant is ground off so that the epoxy resin remains in the cavities and the surface is as flat as possible. To achieve high contrast between the resin and the aggregates/bitumen, fluorescent pigments excited by UV light have proven effective.
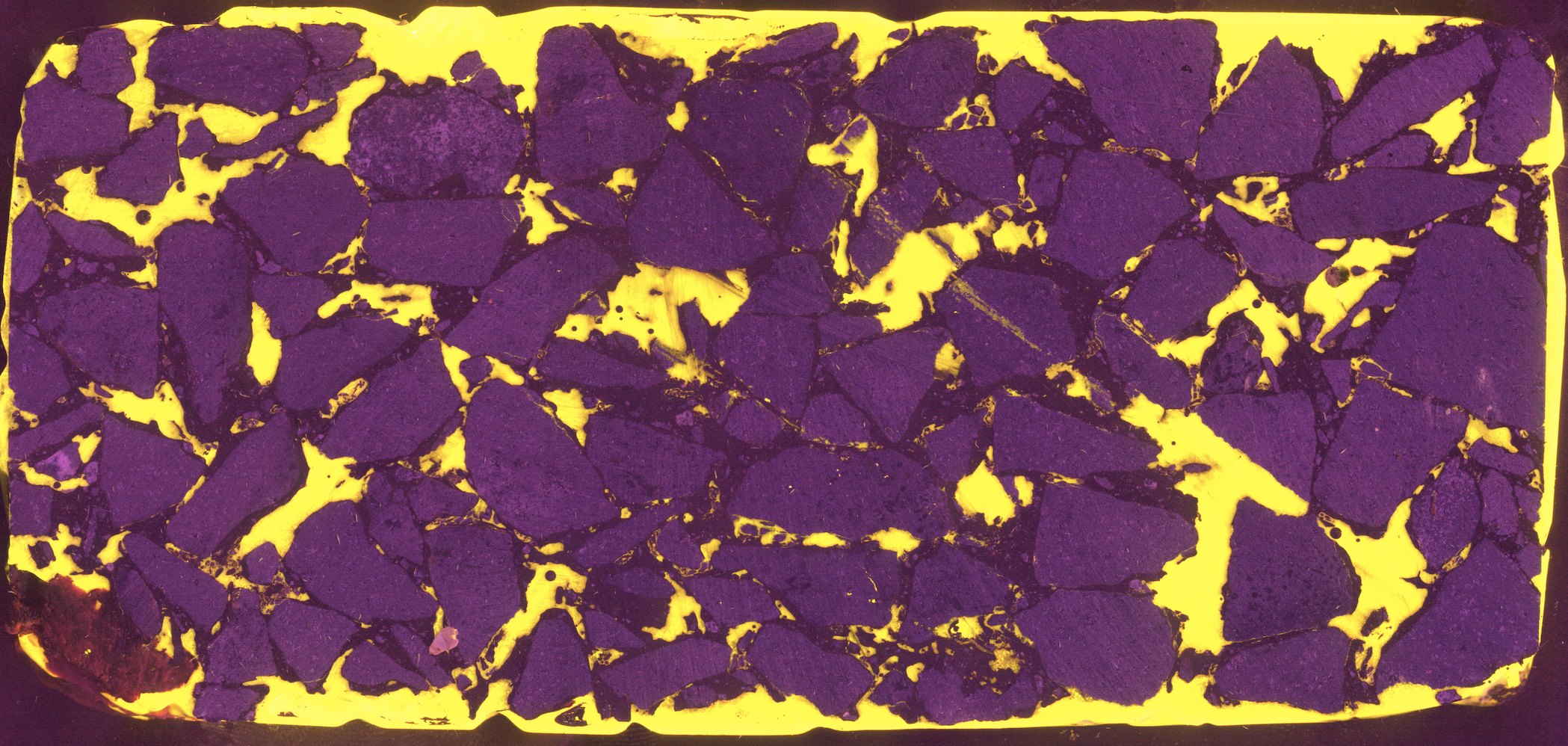
The finished specimen (cf. Figure 1) is scanned using a flatbed scanner equipped with UV LEDs. The high-resolution image is subsequently analysed using the open-source software JMicroVision [45]. The fluorescent voids can be delineated from the rest of the asphalt using colour thresholding. The individual void objects are generated by an object recognition algorithm based on contiguous pixels classified as voids. The software calculates various geometric data of the objects that can be exported for further processing. A more detailed description of the preparation and evaluation method can be found in [20]. As noted in section section 1.1, asphalt petrology can be used for various applications.
2. Laboratory Program
Figure 2 shows the laboratory program investigating possible relationships between void classes, area voids, and permeability of asphalt specimens. A grey shade indicates a process of mix preparation, red indicates specimen preparation, and blue indicates testing.
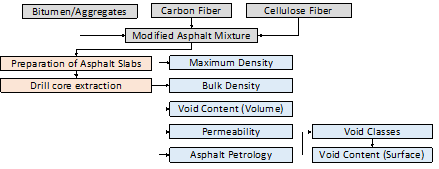
During permeability tests water streams through the asphalt specimen to mimic the real-life use case. Therefore, it is necessary to modify the PA 16 to enhance its durability and temperature conductivity while maintaining a sufficient level of permeability. To this end, different amounts of carbon and cellulose fibres were added to the basic asphalt mixture. Carbon fibres were used to enhance durability. Cellulose was used to promote the adhesion of bitumen to the aggregate since the mixture contains only a small amount of fine aggregate. The fibre compositions of different asphalt mixtures are depicted in Table 1.
Table 1: Composition of the asphalt mixtures produced
Asphalt Mixture |
Amount of cellulose fiber [%] |
Amount of carbon fiber [%] |
M1 |
0.50 |
0.00 |
M2 |
0.40 |
0.10 |
M3 |
0.30 |
0.20 |
M4 |
0.20 |
0.30 |
M5 |
0.10 |
0.40 |
M6 |
0.00 |
0.50 |
Six different standardised asphalt mixtures PA 16 WDA were produced. Drill cores were taken out of asphalt slabs produced out of these mixtures in the laboratory with a roller compactor.
As a next step, conventional properties such as maximum density, bulk density and volume void content were calculated for the specimens produced. These results are shown in Table 2.
Table 2: Results of the conventional analysis of the characteristic values for the asphalt mixes M1 to M6
Asphalt Mixture |
Maximum density [g/cm3] |
Bulk Density [g/cm3] |
Void Content (Volume) [%] |
M1 |
2.859 |
2.076 |
27.4 |
M2 |
2.706 |
2.103 |
22.3 |
M3 |
2.806 |
2.13 |
24.1 |
M4 |
2.792 |
2.145 |
23.2 |
M5 |
2.719 |
2.105 |
22.6 |
M6 |
2.765 |
2.103 |
24.0 |
The porous asphalt pavement’s effectiveness can be evaluated based on its vertical permeability. Vertical permeability is measured according to the German guideline TP Asphalt-StB, Part 19, which supplements the European standard DIN EN 12697 [38].
The vertical permeability describes the asphalt's ability to transport water from its upper to lower margin. The used test device utilises cylinders and water basins to maintain a constant flow of water through the asphalt specimen (cf. Figure 3). The amount of water that flows through the specimen in a given time is measured in parallel.
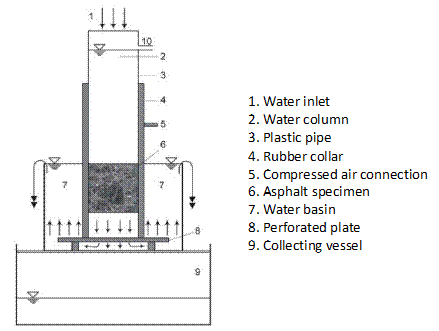
Following the permeability tests asphalt petrological sections were prepared. Void classes were defined based on the scanned sections, and the corresponding void surface area was determined. The void classes were classified according to the information provided in Figure 5.
3. Evaluation of the results
3. 1. Laboratory Results
Figure 4 shows the results of the permeability tests. These are mean values from three individual measurement results in each case. With a permeability value of roughly 1.29 mm/s, the asphalt mixes M1 and M3 show the highest permeability. The mean standard deviation for the asphalt mixes M1 to M6 is 0.059 mm/s. Since multiple aggregates larger than 11.2 mm were used, there is a minimal amount of mastic or finely granulated material inside the mixture, which would generally lead to homogenisation of the whole material. Therefore, although the void content might be the same across the mixtures, voids in the specimens can differ in their composition, size and tortuosity leading to variations in permeability. Since the same drill cores were used for the permeability and asphalt petrological tests, these variations occur at equal rates.
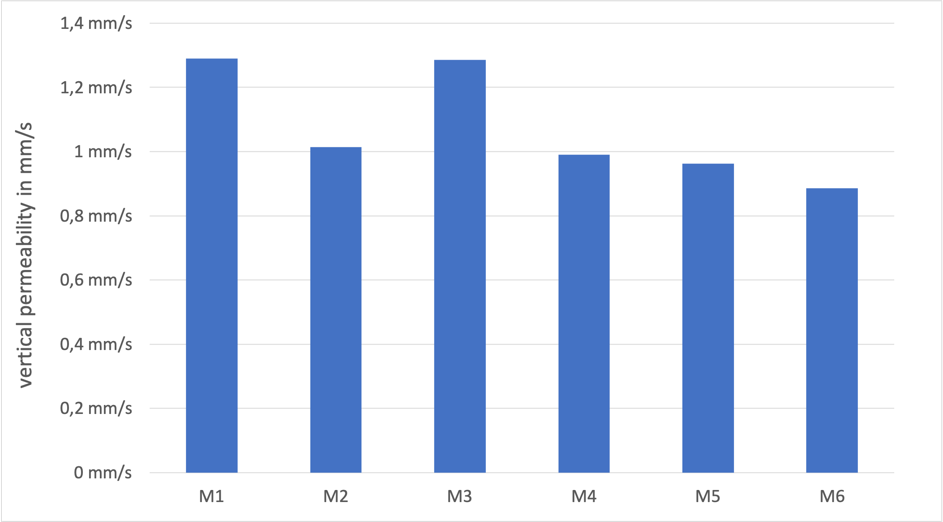
According to DIN EN 12697 [38], which forms the basis of the described permeability test, a permeability value of 0.5 mm/s to 3.5 mm/s is proposed for porous asphalts in surface layers. Since the values of the porous asphalt are within the given limits, it can be assumed that all asphalt mixtures are suitable for a porous asphalt application.
Masad et al. [33] stated that void content significantly impacts the asphalt's permeability. Therefore, any correlations between void content and permeability and between bulk density and permeability were investigated for the asphalt mixes produced.
As described in section 1.4, asphalt petrology offers the possibility of gaining insights into the internal structure of an asphalt specimen. A microsection represents only a single snapshot of the internal network due to its two-dimensional nature. Therefore, three polished sections were prepared and subsequently evaluated for each asphalt mixture to obtain a more accurate understanding of the asphalt specimen’s void structure (M1 to M6). All data of the void surface area was thus calculated from the arithmetic mean of three polished sections.
To evaluate the individual specimens' void structure in a differentiated manner, the void surface area was divided into six classes, each representing a defined range of void sizes (cf. Figure 5). Class A (the void surface area from 0.05 mm2 to 0.1 mm2) is the smallest class. Voids with an area smaller than 0.05 mm2 were not considered in the evaluation.
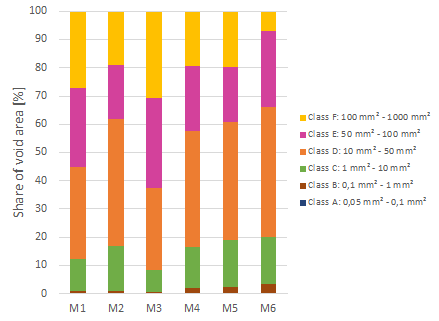
Figure 5 shows that at an average of 39.23 %, the mid-level class D voids make up the largest share of the void surface area. In contrast, class A voids are significantly less common, with an average of 0.024 %. The following section examines the share of void classes and their potential correlations to the permeability of the respective asphalt mix types. The overall standard deviation is within the range of 2.31 % and 3.54 %.
3. 2. Correlation study
Singular linear regressions were used to determine potential correlations between the selected asphalt properties and permeability using equation (3).

FR = Permeability [mm/s]
VC = Void Content [%]
β1,β2 = Regression Constants [-]
First, the potential correlation between bulk density and void content was investigated. The graphs resulting from the single linear regression are shown in Figure 6 as red dotted lines. The coefficient of determination for the void content was calculated as R2=0.0326. Consequently, no correlation could be demonstrated.
Next, an investigation into the potential correlation between flow permeability and void content produced a coefficient of determination of R2=0.4577. Thus, only a poor correlation can be identified here at best. A simplified description of the relationship between volume void content and permeability via a singular linear regression based on the more complex method of Masad et al. [41] was not possible.
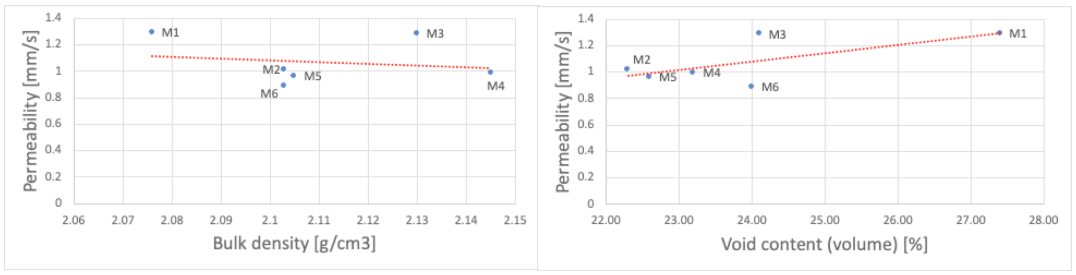
The next step investigated any potential correlation between the respective void surface area of the defined classes (cf. Figure 5) and the permeability. To this end, equation (3) was also applied. The resulting regression graphs for void classes A to F are shown in Figure 7 as red dotted lines. Of note, high coefficients of determination (above 75 %) are obtained for area void classes B, C, D and F (cf. Table 3). Class A was considered negligible since area voids below 0.1 mm2 should play a minor role in permeability. Furthermore, the total share of class A voids is very low.
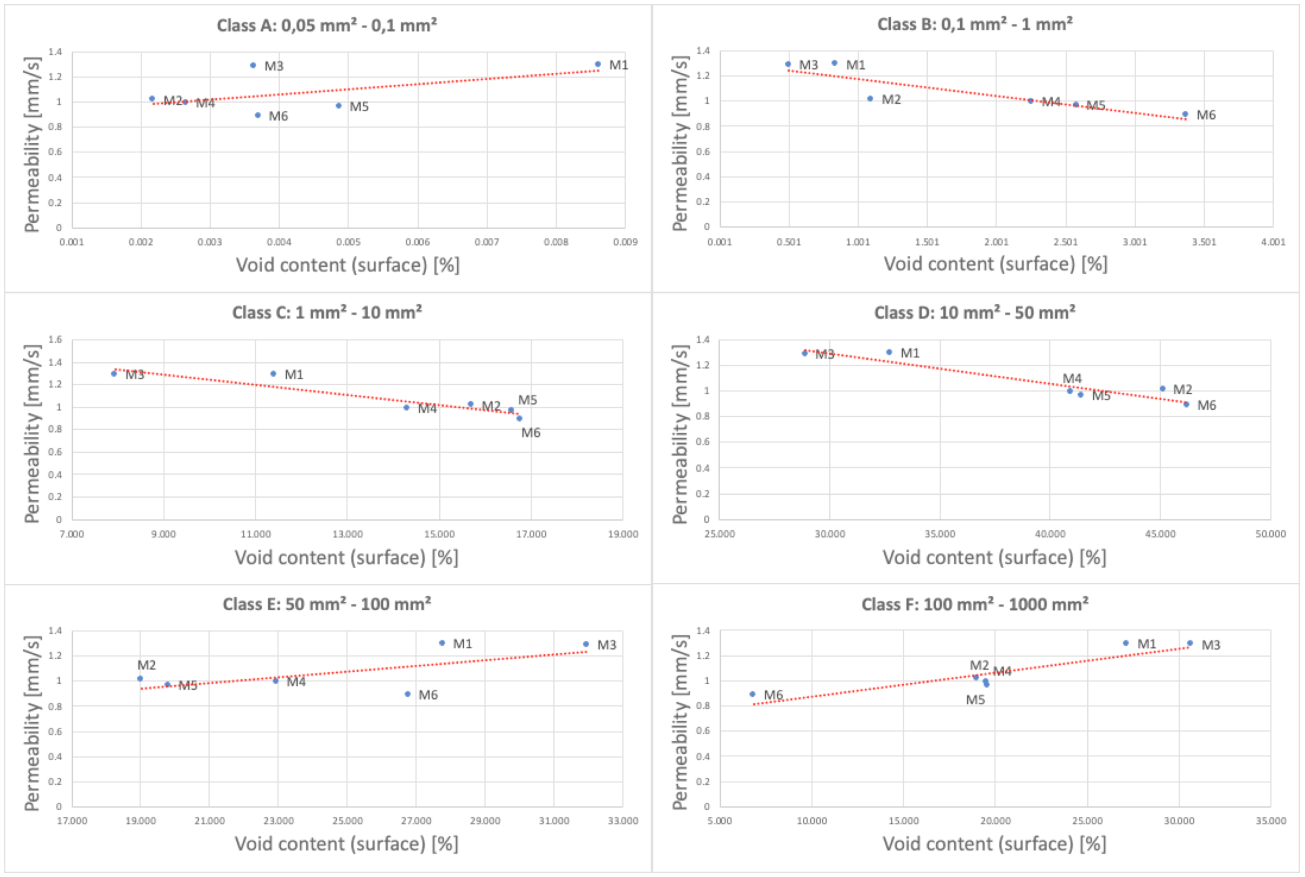
For the smaller void classes B, C and D, an inversely proportional relationship is observed where the gradient of the regression line is negative. As the share of void surface area increases, the f permeability decreases, which sounds contradictory at first. However, the increase in the void surface area of the smaller classes B, C, and D means that the void area content increases in the larger void classes E and F. For these larger classes, there is a proportional relationship to permeability where the gradient of the regression line is positive. It can be concluded that larger void classes are most important in assessing permeability.
Table 3: Results for the coefficient of determination from the single linear regression (void surface area content/permeability) for asphalt mixes M1 to M6
Void Surface Area Class |
Individual coef. of Determination (R2) |
A |
0.304 |
B |
0.774 |
C |
0.855 |
D |
0.885 |
E |
0.430 |
F |
0.816 |
The relationship between permeability and different void classes suggested that a function needs to consider all relevant void classes to be useful. A multiple linear regression was used to investigate the overall correlation between void classes and permeability. Equation (4), which does not contain a regression intercept (unlike equation (3)), is used in this regression. This implies that the function to be determined will intersect the Y-axis at the zero point since the flow rate must be 0 mm/s for a total void content of 0 %. Void class A was excluded from the regression analysis for the reasons detailed in the previous section. The results of the regression coefficients can be found in Table 4.

The more independent variables were included in the model, the higher the coefficient of determination. This was independent of whether the additional independent variables contribute to explaining the correlations. Therefore, the adjusted coefficient of determination was used to assess the quality of multiple regression models. In contrast to the simple coefficient of decision, the adjustment considers the number of independent variables in the model. [46]
Table 4: Results of the multiple regression (share of void area/flow rate) for asphalt mixes M1 to M6
Void Surface Area Class |
Regression Constant |
Adjusted Coef. of Determi-nation (R2) |
Significance |
B |
β1=-0.113 |
0.998 |
0.023 |
C |
β2=-0.061 |
||
D |
β3=-0.012 |
||
E |
β4=+0.028 |
||
F |
β5=+0.011 |
The F-test can be performed to see whether the regression model is statistically significant. It tests if the predictive value of the dependent variable is improved by adding the independent variable [47]. At 0.023, the significance value is relatively small and is below the maximum alpha value of 0.050 usually defined for statistical evaluation of test methods [48].
4. Conclusion
This research is the first approach to determine the permeability by analysing the void surface area using asphalt petrology. The asphalt petrological investigations have shown a significant correlation between void surface area and the permeability of a corrugator sample. To test this correlation, it is necessary to differentiate air void surfaces into five to six void classes. Permeability can be assessed more accurately by considering all void classes. As the share of smaller cavity classes decreases, the share of larger cavity classes increases, which in turn increases the permeability of the specimen.
However, these test results apply only to vertical permeability and not to horizontal permeability. Moreover, the tests were carried out only on fibre-modified WPAs under constant temperature conditions. Even though the overall significance of the correlation is acceptable at 0.023, the individual significance values of the regression parameters β1 to β5 with an average of 0.249 indicate room for improvement. Moreover, asphalt petrology only covers a two-dimensional view of the voids. A three-dimensional examination would be possible at high cost only with CT analysis. Therefore, further investigations, increasing the number of samples and studying additional mixture types under different conditions, are recommended. It is conceivable to improve the predictive power of the equation by adding constants that take into account flow direction and temperature, among others. Additional validations of this paper should be carried out using specimens from construction sites to determine possible deviations in field applications.
References
[1] D. Castro-Fresno, V. Andrés-Valeri, L. Sañudo-Fontaneda und J. Rodriguez-Hernandez, "Sustainable Drainage Practices in Spain, Specially Focused on Pervious Pavements," Water, pp. 67-93, 2013. View Article
[2] J. Rodriguez-Hernandez, V. Andrés-Valeri, M. Calzada-Pérez, Á. Vega-Zamanillo und D. Castro-Fresno, "Study of the Raveling Resistance of Porous Asphalt Pavements Used in Sustainable Drainage Systems Affected by Hydrocarbon Spills," Sustainability, Bd. 7, Nr. 12, pp. 16226-16236, 2015. View Article
[3] C.-F. Chen, J.-W. Lin und J.-Y. Lin, "Hydrological Cycle Performance at a Permeable Pavement Site and a Raingarden Site in a Subtropical Region," Land, Bd. 11, Nr. 6, p. 951, 2022. View Article
[4] S. Alber, W. Ressel, P. Liu, J. Hu, D. Wang, M. Oeser, D. Uribe und H. Steeb, "Investigation of microstructure characteristics of porous asphalt with relevance to acoustic pavement performance," International Journal of Transportation Science and Technology, Bd. 7, Nr. 3, pp. 199-207, 2018. View Article
[5] A. Gupta, J. Rodriguez-Hernandez und D. Castro-Fresno, "Incorporation of Additives and Fibers in Porous Asphalt Mixtures: A Review," Materials, Bd. 12, Nr. 19, p. 3156, 2019. View Article
[6] M. Rodríguez-Rojas, F. Huertas-Fernández, B. Moreno, G. Martinez und A. L. Grindlay, "A study of the application of permeable pavements as a sustainable technique for the mitigation of soil sealing in cities: A case study in the south of Spain," Journal of Environmental Management, Bd. 205, 2018. View Article
[7] K. Kumar, J. Kozak, L. Hundal, A. Cox, H. Zhang und T. Granato, "In-situ infiltration performance of different permeable pavements in a employee used parking lot - A four-year study," Journal of Environmental Management, Bd. 167, pp. 8-14, 2016. View Article
[8] P. Rungruangvirojn und K. Kanitpong, "Measurement of visibility loss due to splash and spray: porous, SMA and conventional asphalt pavements," International Journal of Pavement Engineering, Bd. 11, Nr. 6, pp. 499-510, 2010. View Article
[9] W. B. King Jr., M. S. Kabir, S. B. Cooper Jr. und C. Abadie, "Evaluation of Open Graded Friction Course (OGFC) Rep. FHWA/LA 13/513," Louisiana Transp. Res. Cent., Baton Rouge, LA, 2013.
[10] D. A. Rand, "Permeable Friction Courses TxDOT Experiences," Baton Rouge, LA, 2004.
[11] L. Moretti, G. Cantisani, M. Carpiceci, A. D'Andrea, G. Del Serrone, P. Die Mascio und G. Loprencipe, "Cool pavements are reflective and/or permeable pavements that improve microclimate of urban areas where heat islands cause discomfort to citizens. Stone pavements lower surface temperatures and reduce the amount of heat absorbed. This study assessed, usin," International Journal of Environmental Research and Public Health, Bd. 18, Nr. 24, p. 13108, 2021. View Article
[12] M. Santamouris, "Using cool pavements as a mitigation strategy to fight urban heat island-A review of the actual developments.," Renewable Sustainable energy revews, Bd. 26, pp. 224-240, 2013. View Article
[13] M. V. Akpinar und S. Sevin, "Reducing Urban Heat Islands by Developing Cool Pavements," in The Role of Exergy in Energy and the Environment, S. Nizetic und A. Papadopoulos, Hrsg., Cham, Switzerland, Green Energy and Technology; Springer, 2018. View Article
[14] U.S. Evironmental Protection Agency, "Recuging Urban Heat Islands Compendium Strategies: Cool Pavements," U.S. Evironmental Protection Agency, Washington, DC, 2012.
[15] P. Pascual-Munoz, D. Castro-Fresno, P. Serrano-Bravo und A. Alonso-Estebanez, "Thermal and hydraulic analysis of multilayered asphalt pavements as active solar collectors," Applied Energy, Bd. 11, pp. 324-332, 2014. View Article
[16] K. Schindler, Untersuchung des Verformungsvrhaltens von Asphalt zur Bestimmung von Materialkennwerten für die Dimensionierung., Braunschweig: Technische Universität Carolo-Wilhelmina zu Braunschweig, 2008.
[17] D. C. Wijeyesekera, N. A. R. B. M. Nazari, S. M. Lim, M. I. M. Masirin, A. Zainorabidi und J. Walsh, "Investigation into the Urban Heat Island Effects from Asphalt Pavements," OIDA International Journal on Sustainable Development, Bd. 5, pp. 97-118, 2012.
[18] S. Sodoudi, P. Shahmohamadi, K. Vollack, U. Cubasch und A. I. Che-Ani, "Mitigating the Urban Heat Island Effect in Megacity Tehran," Advances in Metrology, Nr. Special Issue, pp. 1-19, 2014. View Article
[20] M. R. D. Tielmann und T. J. Hill, "Air Void Analyses on Asphalt Specimens Using Plane Section Preparation and Image Analysis," Journal of Materials in Civil Engineering, Bd. 30, Nr. 8, pp. 04018189-1 - 04018189-11, 2018. View Article
[21] M. A. Hernandez-Saenz, S. Caro, E. Arámbula-Mercado und A. Epps Martin, "Permeable Friction Courses (PFC) are asphalt mixtures characterized by high air void contents that are used as surface layers in conventional pavements, principally to achieve safer roads and control noise pollution. This paper summarizes existing informa," Construction and Building Materials, Bd. 111, pp. 358-367, 2016. View Article
[22] S. M. Abtahi, M. Sheikhzadeh und S. M. Hejazi, "Fiber-reinforced asphalt-concrete - A review," Construction and Building Materials, Bd. 24, pp. 871-877, 2010. View Article
[23] K. Krayushkina, O. Prentkovskis, A. Bieliatynskyi, J. Gigineishvili, A. Skrypchenko, A. Laurinavičius, K. Gopalakrishnan und J. Tretjakovas, "Perspectives on using basalt fiber filaments in the construction and rehabilitation of highway pavements and airport runways," The Baltic Journal of Road and Bridge Engineering, Bd. 11, Nr. 1, pp. 77-83, 2016. View Article
[24] S. Wu, Q. Ye, N. Li und H. Yue, "Effects of fibers on the dynamic properties of asphalt mixtures," Journal of Wuhan University of Technology-Mater. Sci. Ed., Bd. 22, Nr. 4, pp. 733-736, 2007. View Article
[25] J.-S. Chen und K.-Y. Lin, "Mechanism and behavior of bitumen strength reinforcement using fibers," Journal of Materials Science, Bd. 40, Nr. 1, pp. 87-95, 2005. View Article
[26] K. R. Lyons und B. J. Putman, "Laboratory evaluation of stabilizing methods for porous asphalt mixtures," Construction and Building Materials, Bd. 49, pp. 772-780, 2013. View Article
[27] M. L. Afonso, M. Dinis-Almeida und C. S. Fael, "Study of the porous asphalt performance with cellulosic fibres," Construction and Building Materials, Bd. 135, pp. 104-111, 2017. View Article
[28] T. Geckil und P. Ahmedzade, "Effects of Carbon Fibre on Performance Properties of Asphalt Mixtures," The Baltic Journal of Road and Bridge Engineering, Bd. 15, Nr. 2, pp. 49-65, 2020. View Article
[29] X. Liu und S. Wu, "Study on the graphite and carbon fiber modified asphalt concrete," Construction and Building Materials, Bd. 25, Nr. 4, pp. 1807-1811, 2011. View Article
[30] Z. Wang, J. Gao, T. Ai und P. Zhao, "Laboratory Investigation on Microwave Deicing Function of Micro Surfacing Asphalt Mixtures Reinforced by Carbon Fiber," Journal of Testing and Evaluation, Bd. 42, Nr. 2, 2014. View Article
[31] K. Zhang, J. Lim, S. Nassiri, K. Englund und H. Li, "Reuse of carbon fiber composite materials in porous hot mix asphalt to enhance strength and durability," Case Studies in Construction Materials, Bd. 11, 2019. View Article
[32] S. Böhm, P. Breitbach, V. Root und V. Wallrabenstein, Offene Asphalte, Teil I: Wasserdurchlässiger Asphalt, Bonn: Deutscher Asphaltverband e. V., 2007.
[33] ASTM PS129-01 Standard provisional test method for measurement of permeability of bituminous paving mixtures using a flexible wall permeameter, ASTM International, 2001.
[34] L. N. Mohammad, A. Herath und B. Huang , "Evaluation of Permeability of Superpav® Asphalt Mixtures," Transportation Research Record: Journal of the Transportation Research Board, Bd. 1832, Nr. 1, pp. 50-58, 2003. View Article
[35] E. Vivar und J. Haddock, "Hot-mix asphalt permeability and porosity," Asphalt Paving Technology: Association of Asphalt Paving Technologists-Proceedings of the Technical Sessions, Bd. 76, pp. 953-979, 2007.
[36] A. E. Alvarez, A. E. Martin und C. Estakhri, "Drainability of Permeable Friction Course Mixtures," Journal of Materials in Civil Engineering, Bd. 22, Nr. 6, pp. 556-564, 2010. View Article
[37] A. J. Cooley Jr., "PERMEABILITY OF SUPERPAVE MIXTURES: EVALUATION OF FIELD PERMEAMETERS," National Center for Asphalt Technology , Auburn Univeristy, Alabama, 1999.
[38] FGSV, TP Asphalt-StB - Teil 19: Durchlässigkeit von Asphalt-Probekörpern, Köln: FGSV Verlag, 2009.
[39] A. L. Cooley Jr. und R. E. Brown, "Selection and Evaluation of Field Permeability Device for Asphalt Pavements," Transportation Research Record, Bd. 1723, Nr. 1, pp. 73-82, 2000. View Article
[40] A. Alomari, L. Tashman, E. Masad, A. L. Cooley Jr. und T. Harman, "Proposed Methodology for Predicting HMA Permeability," Asphalt Paving Technology: Association of Asphalt Paving Technologists-Proceedings of the Technical Sessions, Bd. 71, pp. 30-58, 2002.
[41] E. Masad, A. Al-Omari und R. Lytton, "Simple Method for Predicting Laboratory and Field Permeability of Hot-Mix Asphalt," Transportation Research Record: Journal of the Transportation Research Board, Bd. 1970, Nr. 1, pp. 55-63, 2006. View Article
[42] J. B. Król, R. Khan und A. C. Collop, "The study of the effect of internal structure on permeability of porous asphalt," Road Materials and Pavement Design, Bd. 19, Nr. 4, pp. 935-951, 2018. View Article
[44] K. Eriksen, V. Wegan und J. Karup, "Air void content and other air characteristics of asphalt concrete by image analysis," in Strategic Highway Research Program, Washington, DC, 1992.
[45] N. Roduit, JMicroVision: Image analysis toolbox for measuring and quantifying components of high-definition images. (1.3.4), 2020.
[46] M. Kühlmeyer und C. Kühlmeyer, M. Kühlmeyer, Statistische Auswertungsmethoden für Ingenieure. 2001, Heidelberg: Springer, 2001. View Article
[47] L. Harries and S. Böhm, “Influence of the Shear Process on the Compaction Success and Friction Behavior During Asphalt Paving,” Int. J. Pavement Res. Technol., Jan. 2023, doi: 10.1007/s42947-022-00265-9. View Article
[48] FGSV, Merkblatt über die statistische Auswertung von Prüfergebnissen - Teil 1: Grundlagen zur Präzision von Prüfver- fahren, Bd. 730 Ausgabe 2005, Köln: FGSV Verlag, 2000. View Article