Volume 7 - Year 2024- Pages 194-200
DOI: 10.11159/ijci.2024.020
Evaluating the Impact of Specimen Shape and Size on the Strength Characteristics of Recycled Aggregate Concrete
Yahya Salah1, Karol Sikora1, Kamal Jaafar 1, Sana Amir1
1University of Wollongong in Dubai
Knowledge Village, Dubai, United Arab Emirates
yahyasalah@uowdubai.ac.ae; karolsikora@uowdubai.ac.ae;
kamaljaafar@uowdubai.ac.ae; sanaamir@uowdubai.ac.ae
Abstract - The use of green concrete is increasing worldwide. The growing global demand for utilizing recycled materials in concrete mixes to enhance sustainability makes it important to study the strength properties and behaviour of recycled aggregate concrete (RAC). While the impact of specimen shape and size on the compressive and flexural strength of normal concrete is well-documented, their effects on concrete mixes containing recycled aggregate and ceramic waste are not yet defined. This paper studies the effect of specimen shape and size on the strength properties of RAC by conducting compression tests on cubes and cylinders and performing four-point flexural tests on beams. Locally available recycled coarse aggregate, ceramic fine aggregate, and ceramic waste powder were used to develop the recycled aggregate concrete. Compression tests were conducted on standard cubes, standard cylinders, and half-scale cylinders. Flexural tests were performed on beams sized 150 mm×150 mm×460 mm and 75 mm×75 mm×230 mm. Based on the experimental results, it was found that the specimen shape and size significantly affect the strength properties of RAC, and the conversion factors differ from those of normal concrete with the same strength. Additionally, a preliminary relationship between the compressive strength and flexural strength of RAC is suggested.
Keywords: Green concrete, Ceramic waste material, Size effect, Sustainability, Strength properties.
© Copyright 2024 Authors - This is an Open Access article published under the Creative Commons Attribution License terms. Unrestricted use, distribution, and reproduction in any medium are permitted, provided the original work is properly cited.
Date Received: 2024-05-31
Date Revised: 2024-10-19
Date Accepted: 2024-11-02
Date Published: 2024-12-10
1. Introduction
In recent years, the construction industry has been blooming, and subsequently, the demand for concrete has rocketed. As a result, concerns regarding the impact of concrete on the environment and natural resources started increasing. The main constituent of concrete is the ordinary Portland cement (OPC). The production of OPC is responsible for 5-7% of the CO2 global emissions [1]. Moreover, the demand for natural sand and aggregate reached 32-50 billion tonnes annually [2], a rate that exceeds the natural renewal capacity and can result in sand and aggregate supply shortage by mid-century [3]. On the other hand, construction and demolition (C&D) waste produced annually increases the burden on the environment [4]. For the mentioned reasons, the research to use recycled construction material and reduce the cement in concrete mixes to produce greener concrete has arisen globally over the past years [5-7]. Studies investigated the use of alternative cementitious materials such as fly ash and ground granulated blast furnace slag (GGBFS) [8], biochar [9], or the use of recycled waste materials such as waste glass [10], plastic [11], and recycled aggregate from construction waste [12]. However, advancements in structural engineering research continue [13-16] with reinforced concrete remaining the primary material.
Recycled aggregate (RA) is produced through crushing, cleaning, and screening of C&D waste, and then used again in fresh concrete mixes [17]. Many authors investigated the use of recycled aggregate concrete (RAC) in Ordinary Portland Cement Concrete (OPCC). Most researchers reported a decrease in concrete quality as the natural coarse aggregate replacement ratio with RA increased. It was reported that the use of RA resulted in a lower workability of fresh concrete [18] and 5%-15% more water was needed to achieve the same workability as the 100% natural aggregate concrete (NAC) which is attributed to the increased water absorption of recycled aggregate [19]. Moreover, the utilization of RA usually results in a lower compressive strength, in particular, if the replacement ratio of natural aggregate with RA is 100%, the drop in compressive strength can reach up to 20-25% [20]. Moreover, RA has a negative effect on the elastic modulus of concrete [21]. However, studies were carried out to investigate enhancing the bond between the RA and the cement mix by treating the RA surface using different techniques [17], [22], and acceptable [23] and high-performance mixes [24] were developed using RA.
Compressive and flexural strength are the most important mechanical properties of concrete and are crucial in the designing of concrete structures. Compressive strength can be obtained by testing standard cylinders and cubes under compressive forces until failure [25]. On the other hand, flexural strength is assessed by testing of beam specimen subject to a four-point or three-point bending test [26]. At times, an estimate of the flexural strength can be obtained by using the established relationship between the compressive strength and the flexural strength. The most common form of this relation is:
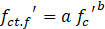
Where 𝑓ct.f′ is the flexural tensile strength in MPa, 𝑓𝑐′ is the compressive strength in MPa, and “a” and “b” are factors and different codes have different values for ‘a’ and ‘b’. For instance, the AS 3600:2018 suggests that a = 0.6 and b = ½ [27] while ACI 318-19 suggests that a = 0.62 and b = ½ [28]. However, further investigation is needed to check if this relationship is affected by the change in the type of aggregate or cement.
However, the strength properties of concrete are not unique and they are affected by the change in some factors including the size and the shape of the sample, generally, cubes show higher strength than cylinders, and smaller samples show higher strength than larger samples [29-32]. In the AS 3600:2018 for instance, a conversion factor of 0.8 was given to convert from standard cube compressive strength to standard cylinder compressive strength [27]. However, this is suggested for ordinary concrete reinforced and may not be applied to every type of cement or aggregate as changing the mix materials results in a change in the way concrete behaves. In addition, although some of the standards such BS 8500-2:2023 [25] allow the use of cubes of different sizes and ASTM C39 [33] allows the use of cylinders of different sizes, none of them mention the use of a size factor to convert from one sample size to the other. Moreover, the Australian standards [27] recognize the existence of size effect by limiting the combination of the results from the two cylinder sizes that it uses, however, it does not specify a conversion factor between the two sizes. This suggests the need for more research to be done on the specimen shape and size effect on the concrete mechanical properties, in particular, the more novel concrete mixes such as RAC.
Various studies have been done to investigate the effect of sample size and shape on Recycled Aggregate Concrete (RAC). Some literature reports a cube-to-cylinder conversion factor of 0.78–0.93 for RAC, with no size effect observed [34]. In another study [35], the cube-to-cylinder conversion factor for RAC, using 200 cylinders and cubes, was found to be 0.7. The size effect was also examined for RAC in cubes [36] and cylinders [37], both of which reported a negative size effect, indicating greater strength with larger samples. However, none of these studies tested the size effect on flexural strength, nor did they utilize 100% recycled aggregate or ceramic waste materials in their concrete mixes.
This paper aims to examine the effect of specimen size and shape on the mechanical properties (compressive and flexural strength) of recycled aggregate concrete while utilizing 100% recycled coarse aggregate and ceramic waste materials.
2. Experimental Setup
2. 1. Concrete Mix
The concrete mix used in this study was developed by Batikha et al. to investigate the use of recycled coarse aggregate and ceramic waste materials and their effect on the strength properties of concrete [38]. In this mix, 100% of the natural coarse aggregate was replaced with recycled aggregate, 20% of the natural fine aggregate was replaced with ceramic fine aggregate, and 20% of Portland cement was replaced with ceramic waste powder. The mix was designed to achieve a targeted characteristic cylindrical compressive strength of 40 MPa and a mean cylindrical compressive strength of 40 MPa [38]. The same mix was adopted with a slight increase in the water-to-cement ratio from 0.4 to 0.5. The mix design is shown in Table 1.
Table 1. Concrete Mix Design.
Material |
Quantity/m3 |
Material |
Quantity/m3 |
Liter (L) / Kilogram (kg) |
Liter (L) / Kilogram (kg) |
||
OPC |
320 kg |
Natural fine aggregate |
796 kg |
Ceramic Waste Powder |
80 kg |
Ceramic fine aggregate |
199 kg |
Recycled Coarse Aggregate |
827 kg |
Water |
160 L |
Super-plasticizer |
5 L |
2. 2. Materials
The cement used in this study is Type I Ordinary Portland Cement class 42.5 N. Black sand and ceramic fine aggregate with a maximum particle size of less than 5 mm were used as fine aggregate. Moreover, Recycled Coarse Aggregate (RCA) with a maximum particle size of 14 mm was obtained from Beea’h Recycling factory. The water absorption for recycled aggregate is 4.8%. The ceramic waste powder (CWP) used in this study passed the 125-micrometer sieve. The superplasticizer used in this study is poly-carboxylate ether with the commercial name of PC400 supplied by PAC technology.
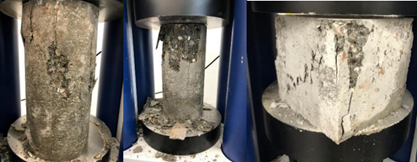
2. 3. Sample Type and Size
Samples of various shapes and sizes were tested, including standard-size cubes of 150×150×150 mm and standard size cylinders measuring 150×300 mm, and half-scale samples measuring 75×150 mm. For each size, nine samples were cast. Three samples of each size were subject to compressive strength testing on the 7th, 14th, and 28th days (Figure 1). Beam sizes of 150×150×460 mm and half scale size of 75×75×230 mm, with three samples cast for each size, were tested for flexural strength using the four-point bending test on the 28th day. Table 2 provides a summary of the specimen, test specifications, and age of testing.
Table 2. Type of specimen and age at test.
Test |
Specimen shape and size |
No. of specimens |
Age |
Days |
|||
Compressive Strength |
150×150×150 mm Cubes |
9 |
7, 14, 28
|
75×150 mm Cylinders |
9 |
||
150×300 mm Cylinders |
9 |
||
Flexural Strength |
150×150×460 mm Beams |
3 |
28 |
75×75×230 mm Beams |
3 |
3. 3 Results and Discussion
3. 1. Effect of sample shape on compressive strength
The effect of specimen shape on compressive strength is evident in the results (see Table 3), as the 150×300 mm cylinders showed lower compressive strength compared to the 150×150×150 mm cubes. An average conversion factor of approximately 0.5 was determined to convert from the standard cube size to the standard cylinder size across all curing ages. This is lower than the provisions of AS 3600 [27] where the average conversion factor is around 0.8. This difference may be attributed to the lower content of Ordinary Portland Cement (OPC) in the mix, as it was partially replaced by ceramic waste powder. Figure 2(a) shows development of compressive strength of cubes with regards to curing age.
Table 3. Experimental results.
Sample shape /type |
Sample size |
Mean strength at 28 days |
Standard deviation |
Coefficient of variation |
mm |
MPa |
MPa |
||
Cubes |
150×150×150 |
61.6 |
3.82 |
6.2 |
Cylinders |
150×300 |
30 |
2.1 |
6.9 |
75×150 |
39.9 |
1.48 |
3.71 |
|
Beams |
150×150×460 |
4 |
0.75 |
18.92 |
75×75× 230 |
6.4 |
0.21 |
3.23 |
3. 2. Effect of Size on Compressive Strength
The small-scale cylinders (75×150 mm), which are half the size of the standard 150×300 mm cylinders, showed higher strength than the standard-size cylinders, as shown in Table 3. This is expected due to the size effect as discussed in section 1. The size factor to convert from half-scale cylinders to standard-size cylinders is 0.78, indicating the effect of size on compressive strength. This size factor is not constant across the curing age (in days) as can be seen in Figure 2(b). Figure 3(a) illustrates the strength development of the different shapes and sizes of concrete samples. The effect of size on compressive strength needs further investigation by testing more sizes for both cylinders and cubes. Another aspect that needs further deliberation is whether using a smaller size aggregate for smaller samples will reduce the size effect or not [39]. It was observed in a finite element study [39], that using a smaller aggregate size reduced the effect of size as it reduced the fracture energy.
3. 3. Effect of Size on Flexural Strength
The impact of size on flexural strength was evident in this experiment. The smaller 75×75×230 mm beams, which are half the size of the 150×150×460 mm beams, displayed a higher flexural strength of 6.4 MPa compared to 4 MPa. The size conversion factor from small to large beams is 0.625. Flexural strength results from study [29] can be utilized to further analyse the size effect. Despite being cured for 56 days, the 100×100×500 mm beam used in that study exhibited lower strength (4.6 MPa) than the 75×75×230 mm beams (6.4 MPa). Additionally, the 100×100×500 mm beam had a smaller width and depth, and it showed higher flexural strength than the large beams in this study (4 MPa). Size factors for converting from the 100×100×500 mm beam tested in Batikha et al. [38] to the small and large beams tested in this study can be 1.36 and 0.87, respectively. However, these size factors might not be extremely accurate as they do not account for differences in W/C ratios and curing ages. Figure 3(b) illustrates the size effect on flexural strength (including the beams from [38]). As mentioned in section 3.2, it would be interesting to reduce the aggregate size for smaller beams and then observe whether any size effect is present [39].
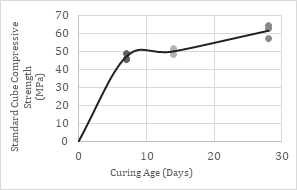
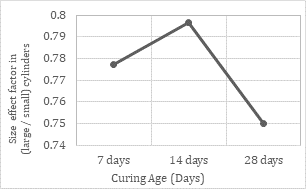
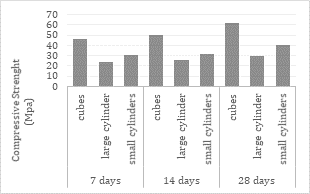
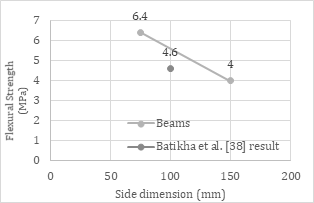
3. 4. Relationship between Compressive and Flexural Strength
The results of the standards cylinder and large beams obtained in this study suggest the a, and b factors in Eq. 1 to be 0.74 and 0.5 respectively, making the final Eq. 2:
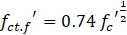
However, this is a preliminary equation and further testing on a greater number of samples needs to be done to confirm the accuracy of this equation. This relationship also needs to be investigated for various types of mixes, for example, different design strengths or for other types of green concrete mixes using recycled aggregate.
4. Conclusion
This study investigated the effect of the specimen size and shape on the compressive and flexural strength of recycled aggregate concrete. Samples of various configurations were cast: nine standard cubes, nine standard and half-scale cylinders, and three beams of large size and half-scale were prepared and water-cured. Three cubes and cylinders of each size were tested for compressive strength after 7, 14, and 28 days, while beams were tested for flexural strength after 28 days. The following conclusions were drawn:
- The average cylinder/cube ratio was found to be 0.5.
- The effect of the sample size was observed on half and full-scale cylinders. A size factor of 0.78 was developed to convert from small to large cylinders.
- The size effect was present in the flexural strength of the beams as well. A size factor of 0.625 was found to convert from small to large beams.
- Lastly, a preliminary relationship between compressive cylinder strength and flexural strength of concrete large beams was suggested, though more testing needs to be done to confirm the viability of the equation.
Acknowledgments
The authors acknowledge the internal research grant provided by the University of Wollongong in Dubai for this work. In addition, the authors would like to acknowledge Bee’ah factory and PAC technology for supplying the material for this research.
References
[1] C. McLellan, R. P. Williams, J. Lay, A. Van Riessen, and G. D. Corder, “Costs and carbon emissions for geopolymer pastes in comparison to ordinary portland cement,” J. Clean. Prod., vol. 19, no. 9–10, pp. 1080–1090, Jun. 2011, doi: 10.1016/j.jclepro.2011.02.010. View Article View Article
[2] L. Koehnken and M. Rintoul, “Impacts of sand mining on ecosystem structure, process and biodiversity in rivers,” World Wildl. Fund Int., vol. 159, 2018.
[3] M. Bendixen, J. Best, C. Hackney, and L. Lonsmann Iversen, “Time is running out for sand,” nature. Accessed: May 18, 2024. [Online]. Available: https://www.nature.com/articles/d41586-019-02042-4#ref-CR3
[4] United States Environmental Protection Agency, “Sustainable Management of Construction and Demolition Materials.” Accessed: May 18, 2024. [Online]. Available: https://www.epa.gov/smm/sustainable-management-construction-and-demolition-materials
[5] A. J. Klemm and K. Sikora, “The effect of cement type on the performance of mortars modified by superabsorbent polymers,” presented at the Concrete Repair, Rehabilitation and Retrofitting III - Proceedings of the 3rd International Conference on Concrete Repair, Rehabilitation and Retrofitting, ICCRRR 2012, 2012, pp. 210–216. [Online]. Available: https://www.scopus.com/inward/record.uri?eid=2-s2.0-84867378368&partnerID=40&md5=f17b76398e2a7b24d400796663cec891
[6] A. J. Klemm, P. Baker, and K. Sikora, “The effect of super absorbent polymers on the performance of immature cementitious mortars,” in Brittle Matrix Composites 10, Elsevier, 2012, pp. 21–31. doi: 10.1533/9780857099891.21. View Article
[7] K. S. Sikora and A. J. Klemm, “The effect of superabsorbent polymers on performance of fly ash cementitious mortars exposed to accelerated freezing/thawing conditions,” Int. J. Comput. Methods Exp. Meas., vol. 2, no. 3, pp. 255–268, Sep. 2014, doi: 10.2495/CMEM-V2-N3-255-268. View Article
[8] M. N. S. Hadi, H. Zhang, and S. Parkinson, “Optimum mix design of geopolymer pastes and concretes cured in ambient condition based on compressive strength, setting time and workability,” J. Build. Eng., vol. 23, pp. 301–313, May 2019, doi: 10.1016/j.jobe.2019.02.006. View Article
[9] S. Abbas and K. S. Sikora, “The Effect of Biochar on Properties of Sulphate Resisting Concrete,” in Proceedings of 2021 4th International Conference on Civil Engineering and Architecture, vol. 201, T. Kang and Y. Lee, Eds., in Lecture Notes in Civil Engineering, vol. 201. , Singapore: Springer Nature Singapore, 2022, pp. 223–230. doi: 10.1007/978-981-16-6932-3_19. View Article
[10] Y. Qian, M. N. Sheikh, H. Feng, and M. N. S. Hadi, “Use of waste glass as fine aggregate in ambient cured alkali‐activated mortars,” Struct. Concr., vol. 24, no. 3, pp. 4145–4160, Jun. 2023, doi: 10.1002/suco.202200760. View Article
[11] R. Siddique, J. Khatib, and I. Kaur, “Use of recycled plastic in concrete: A review,” Waste Manag., vol. 28, no. 10, pp. 1835–1852, 2008, doi: 10.1016/j.wasman.2007.09.011. View Article
[12] A. Rao, K. N. Jha, and S. Misra, “Use of aggregates from recycled construction and demolition waste in concrete,” Resour. Conserv. Recycle. vol. 50, no. 1, pp. 71–81, Mar. 2007, doi: 10.1016/j.resconrec.2006.05.010. View Article
[13] P. Richard, Z. Liu, T. Descamps, and K. S. Sikora, “Effect of concrete modification on shear of connections for timber–concrete composites,” Proc. Inst. Civ. Eng. - Struct. Build. vol. 173, no. 5, pp. 326–339, May 2020, doi: 10.1680/jstbu.19.00046. View Article
[14] K. Jaafar and K. Sikora, “Analytical confinement model for square section confined with circular ties,” Aust. J. Struct. Eng., vol. 21, no. 3, pp. 218–233, Jul. 2020, doi: 10.1080/13287982.2020.1776584. View Article
[15] K. Jaafar, “Testing the applicability of design code provisions - case of unconventional shear reinforcement,” Aust. J. Struct. Eng., vol. 22, no. 4, pp. 251–265, Oct. 2021, doi: 10.1080/13287982.2021.1958507. View Article
[16] K. Jaafar, “Discrete crack sectional analysis for shear evaluation of RC beams,” Int. J. Struct. Eng., vol. 4, no. 4, p. 295, 2013, doi: 10.1504/IJSTRUCTE.2013.056980. View Article
[17] J. Junak and A. Sicakova, “Concrete Containing Recycled Concrete Aggregate with Modified Surface,” Procedia Eng., vol. 180, pp. 1284–1291, 2017, doi: 10.1016/j.proeng.2017.04.290. View Article
[18] R. Kurda, J. De Brito, and J. D. Silvestre, “Influence of recycled aggregates and high contents of fly ash on concrete fresh properties,” Cem. Concr. Compos. vol. 84, pp. 198–213, Nov. 2017, doi: 10.1016/j.cemconcomp.2017.09.009. View Article
[19] A. M. Wagih, H. Z. El-Karmoty, M. Ebid, and S. H. Okba, “Recycled construction and demolition concrete waste as aggregate for structural concrete,” HBRC J., vol. 9, no. 3, pp. 193–200, Dec. 2013, doi: 10.1016/j.hbrcj.2013.08.007. View Article
[20] M. Etxeberria, E. Vázquez, A. Marí, and M. Barra, “Influence of amount of recycled coarse aggregates and production process on properties of recycled aggregate concrete,” Cem. Concr. Res., vol. 37, no. 5, pp. 735–742, May 2007, doi: 10.1016/j.cemconres.2007.02.002. View Article
[21] C.-Q. Lye, R. K. Dhir, and G. S. Ghataora, “Elastic modulus of concrete made with recycled aggregates,” Proc. Inst. Civ. Eng. - Struct. Build, vol. 169, no. 5, pp. 314–339, May 2016, doi: 10.1680/jstbu.15.00077. View Article
[22] H. Choi, R. Kitagaki, and T. Noguchi, “Effective Recycling of Surface Modification Aggregate using Microwave Heating,” J. Adv. Concr. Technol., vol. 12, no. 2, pp. 34–45, Feb. 2014, doi: 10.3151/jact.12.34. View Article
[23] S. Marinković, V. Radonjanin, M. Malešev, and I. Ignjatović, “Comparative environmental assessment of natural and recycled aggregate concrete,” Waste Manag., vol. 30, no. 11, pp. 2255–2264, Nov. 2010, doi: 10.1016/j.wasman.2010.04.012. View Article
[24] G. Andreu and E. Miren, “Experimental analysis of properties of high performance recycled aggregate concrete,” Constr. Build. Mater. vol. 52, pp. 227–235, Feb. 2014, doi: 10.1016/j.conbuildmat.2013.11.054. View Article
[25] British Standards Institution, Ed., Concrete - complementary British Standard to BS EN 206: Specification for constituent materials and concrete. in British standard, no. BS 8500‑2:2023. London: BSI Standards Limited, 2023.
[26] British Standards Institution, Ed., Testing hardened concrete - Flexural strength of test specimensTesting hardened concrete - Flexural strength of test specimens. in British standard, no. BS EN 12390-5:2019-TC. London: BSI Standards Limited, 2019.
[27] Committee BD-002 (last), Concrete structures. Sydney: Standards Australia Limited, 2018.
[28] 318-19 Building Code Requirements for Structural Concrete and Commentary. American Concrete Institute, 2019. doi: 10.14359/51716937. View Article
[29] S. Amir, C. van der Veen, J. Walraven, and A. de Boer, “Punching Shear in Prestressed Concrete Deck Slabs: A Comprehensive Study,” in SP-357: Punching Shear of Concrete Slabs: Insights from New Materials, Tests, and Analysis Methods, American Concrete Institute, 2023. doi: 10.14359/51738765. View Article
[30] S. Amir, C. Van Der Veen, and A. De Boer, “Experimental and numerical investigation of the effect of size in post-tensioned concrete deck slabs,” Proc. Int. Struct. Eng. Constr., vol. 7, no. 1, Aug. 2020, doi: 10.14455/ISEC.res.2020.7(1).STR-52. View Article
[31] S. Amir, C. Van Der Veen, J. C. Walraven, and A. De Boer, “Bearing Capacity of Transversely Prestressed Concrete Deck Slabs,” Struct. Eng. Int., vol. 30, no. 4, pp. 534–544, Oct. 2020, doi: 10.1080/10168664.2019.1695556. View Article
[32] S. Amir, C. Van Der Veen, J. C. Walraven, and A. De Boer, “Punching Shear in Prestressed Concrete Deck Slabs: Parametric Study,” ACI Struct. J., vol. 116, no. 4, Jul. 2019, doi: 10.14359/51715565. View Article
[33] C09 Committee, “Test Method for Compressive Strength of Cylindrical Concrete Specimens.” ASTM International. doi: 10.1520/C0039_C0039M-18. View Article
[34] S. Yehia, A. Abdelfatah, and D. Mansour, “Effect of Aggregate Type and Specimen Configuration on Concrete Compressive Strength,” Crystals, vol. 10, no. 7, p. 625, Jul. 2020, doi: 10.3390/cryst10070625. View Article
[35] A. H. Buller, Mahboob Oad, and B. A. Memon, “Relationship between Cubical and Cylindrical Compressive Strength of Recycled Aggregate Concrete.” Unpublished, 2018. doi: 10.13140/RG.2.2.28872.78086.
[36] B. A. Memon, M. Oad, A. H. Buller, S. A. Shar, A. S. Buller, and F.-R. Abro, “Effect of Mould Size on Compressive Strength of Green Concrete Cubes,” Civ. Eng. J., vol. 5, no. 5, pp. 1181–1188, May 2019, doi: 10.28991/cej-2019-03091322. View Article
[37] A. H. Buller, A. Memon, Jahanzeb Sodhar, Mahboob Oad, and Irum Naz Sodhar, “EFFECT OF SPECIMEN SIZE ON STRENGTH OF GREEN CONCRETE CYLINDERS.” Unpublished, 2020. doi: 10.13140/RG.2.2.36300.77442.
[38] M. Batikha, S. T. M. Ali, A. Rostami, and M. Kurtayev, “Using recycled coarse aggregate and ceramic waste to produce sustainable economic concrete,” Int. J. Sustain. Eng., vol. 14, no. 4, pp. 785–799, Jul. 2021, doi: 10.1080/19397038.2020.1862353. View Article
[39] S. Amir, “Compressive Membrane Action in Prestressed Concrete Deck Slabs,” PhD thesis, Delft University of Technology, June 2014.
View Article
[1] C. McLellan, R. P. Williams, J. Lay, A. Van Riessen, and G. D. Corder, “Costs and carbon emissions for geopolymer pastes in comparison to ordinary portland cement,” J. Clean. Prod., vol. 19, no. 9–10, pp. 1080–1090, Jun. 2011, doi: 10.1016/j.jclepro.2011.02.010. View Article View Article
[2] L. Koehnken and M. Rintoul, “Impacts of sand mining on ecosystem structure, process and biodiversity in rivers,” World Wildl. Fund Int., vol. 159, 2018.
[3] M. Bendixen, J. Best, C. Hackney, and L. Lonsmann Iversen, “Time is running out for sand,” nature. Accessed: May 18, 2024. [Online]. Available: https://www.nature.com/articles/d41586-019-02042-4#ref-CR3
[4] United States Environmental Protection Agency, “Sustainable Management of Construction and Demolition Materials.” Accessed: May 18, 2024. [Online]. Available: https://www.epa.gov/smm/sustainable-management-construction-and-demolition-materials
[5] A. J. Klemm and K. Sikora, “The effect of cement type on the performance of mortars modified by superabsorbent polymers,” presented at the Concrete Repair, Rehabilitation and Retrofitting III - Proceedings of the 3rd International Conference on Concrete Repair, Rehabilitation and Retrofitting, ICCRRR 2012, 2012, pp. 210–216. [Online]. Available: https://www.scopus.com/inward/record.uri?eid=2-s2.0-84867378368&partnerID=40&md5=f17b76398e2a7b24d400796663cec891
[6] A. J. Klemm, P. Baker, and K. Sikora, “The effect of super absorbent polymers on the performance of immature cementitious mortars,” in Brittle Matrix Composites 10, Elsevier, 2012, pp. 21–31. doi: 10.1533/9780857099891.21. View Article
[7] K. S. Sikora and A. J. Klemm, “The effect of superabsorbent polymers on performance of fly ash cementitious mortars exposed to accelerated freezing/thawing conditions,” Int. J. Comput. Methods Exp. Meas., vol. 2, no. 3, pp. 255–268, Sep. 2014, doi: 10.2495/CMEM-V2-N3-255-268. View Article
[8] M. N. S. Hadi, H. Zhang, and S. Parkinson, “Optimum mix design of geopolymer pastes and concretes cured in ambient condition based on compressive strength, setting time and workability,” J. Build. Eng., vol. 23, pp. 301–313, May 2019, doi: 10.1016/j.jobe.2019.02.006. View Article
[9] S. Abbas and K. S. Sikora, “The Effect of Biochar on Properties of Sulphate Resisting Concrete,” in Proceedings of 2021 4th International Conference on Civil Engineering and Architecture, vol. 201, T. Kang and Y. Lee, Eds., in Lecture Notes in Civil Engineering, vol. 201. , Singapore: Springer Nature Singapore, 2022, pp. 223–230. doi: 10.1007/978-981-16-6932-3_19. View Article
[10] Y. Qian, M. N. Sheikh, H. Feng, and M. N. S. Hadi, “Use of waste glass as fine aggregate in ambient cured alkali‐activated mortars,” Struct. Concr., vol. 24, no. 3, pp. 4145–4160, Jun. 2023, doi: 10.1002/suco.202200760. View Article
[11] R. Siddique, J. Khatib, and I. Kaur, “Use of recycled plastic in concrete: A review,” Waste Manag., vol. 28, no. 10, pp. 1835–1852, 2008, doi: 10.1016/j.wasman.2007.09.011. View Article
[12] A. Rao, K. N. Jha, and S. Misra, “Use of aggregates from recycled construction and demolition waste in concrete,” Resour. Conserv. Recycle. vol. 50, no. 1, pp. 71–81, Mar. 2007, doi: 10.1016/j.resconrec.2006.05.010. View Article
[13] P. Richard, Z. Liu, T. Descamps, and K. S. Sikora, “Effect of concrete modification on shear of connections for timber–concrete composites,” Proc. Inst. Civ. Eng. - Struct. Build. vol. 173, no. 5, pp. 326–339, May 2020, doi: 10.1680/jstbu.19.00046. View Article
[14] K. Jaafar and K. Sikora, “Analytical confinement model for square section confined with circular ties,” Aust. J. Struct. Eng., vol. 21, no. 3, pp. 218–233, Jul. 2020, doi: 10.1080/13287982.2020.1776584. View Article
[15] K. Jaafar, “Testing the applicability of design code provisions - case of unconventional shear reinforcement,” Aust. J. Struct. Eng., vol. 22, no. 4, pp. 251–265, Oct. 2021, doi: 10.1080/13287982.2021.1958507. View Article
[16] K. Jaafar, “Discrete crack sectional analysis for shear evaluation of RC beams,” Int. J. Struct. Eng., vol. 4, no. 4, p. 295, 2013, doi: 10.1504/IJSTRUCTE.2013.056980. View Article
[17] J. Junak and A. Sicakova, “Concrete Containing Recycled Concrete Aggregate with Modified Surface,” Procedia Eng., vol. 180, pp. 1284–1291, 2017, doi: 10.1016/j.proeng.2017.04.290. View Article
[18] R. Kurda, J. De Brito, and J. D. Silvestre, “Influence of recycled aggregates and high contents of fly ash on concrete fresh properties,” Cem. Concr. Compos. vol. 84, pp. 198–213, Nov. 2017, doi: 10.1016/j.cemconcomp.2017.09.009. View Article
[19] A. M. Wagih, H. Z. El-Karmoty, M. Ebid, and S. H. Okba, “Recycled construction and demolition concrete waste as aggregate for structural concrete,” HBRC J., vol. 9, no. 3, pp. 193–200, Dec. 2013, doi: 10.1016/j.hbrcj.2013.08.007. View Article
[20] M. Etxeberria, E. Vázquez, A. Marí, and M. Barra, “Influence of amount of recycled coarse aggregates and production process on properties of recycled aggregate concrete,” Cem. Concr. Res., vol. 37, no. 5, pp. 735–742, May 2007, doi: 10.1016/j.cemconres.2007.02.002. View Article
[21] C.-Q. Lye, R. K. Dhir, and G. S. Ghataora, “Elastic modulus of concrete made with recycled aggregates,” Proc. Inst. Civ. Eng. - Struct. Build, vol. 169, no. 5, pp. 314–339, May 2016, doi: 10.1680/jstbu.15.00077. View Article
[22] H. Choi, R. Kitagaki, and T. Noguchi, “Effective Recycling of Surface Modification Aggregate using Microwave Heating,” J. Adv. Concr. Technol., vol. 12, no. 2, pp. 34–45, Feb. 2014, doi: 10.3151/jact.12.34. View Article
[23] S. Marinković, V. Radonjanin, M. Malešev, and I. Ignjatović, “Comparative environmental assessment of natural and recycled aggregate concrete,” Waste Manag., vol. 30, no. 11, pp. 2255–2264, Nov. 2010, doi: 10.1016/j.wasman.2010.04.012. View Article
[24] G. Andreu and E. Miren, “Experimental analysis of properties of high performance recycled aggregate concrete,” Constr. Build. Mater. vol. 52, pp. 227–235, Feb. 2014, doi: 10.1016/j.conbuildmat.2013.11.054. View Article
[25] British Standards Institution, Ed., Concrete - complementary British Standard to BS EN 206: Specification for constituent materials and concrete. in British standard, no. BS 8500‑2:2023. London: BSI Standards Limited, 2023.
[26] British Standards Institution, Ed., Testing hardened concrete - Flexural strength of test specimensTesting hardened concrete - Flexural strength of test specimens. in British standard, no. BS EN 12390-5:2019-TC. London: BSI Standards Limited, 2019.
[27] Committee BD-002 (last), Concrete structures. Sydney: Standards Australia Limited, 2018.
[28] 318-19 Building Code Requirements for Structural Concrete and Commentary. American Concrete Institute, 2019. doi: 10.14359/51716937. View Article
[29] S. Amir, C. van der Veen, J. Walraven, and A. de Boer, “Punching Shear in Prestressed Concrete Deck Slabs: A Comprehensive Study,” in SP-357: Punching Shear of Concrete Slabs: Insights from New Materials, Tests, and Analysis Methods, American Concrete Institute, 2023. doi: 10.14359/51738765. View Article
[30] S. Amir, C. Van Der Veen, and A. De Boer, “Experimental and numerical investigation of the effect of size in post-tensioned concrete deck slabs,” Proc. Int. Struct. Eng. Constr., vol. 7, no. 1, Aug. 2020, doi: 10.14455/ISEC.res.2020.7(1).STR-52. View Article
[31] S. Amir, C. Van Der Veen, J. C. Walraven, and A. De Boer, “Bearing Capacity of Transversely Prestressed Concrete Deck Slabs,” Struct. Eng. Int., vol. 30, no. 4, pp. 534–544, Oct. 2020, doi: 10.1080/10168664.2019.1695556. View Article
[32] S. Amir, C. Van Der Veen, J. C. Walraven, and A. De Boer, “Punching Shear in Prestressed Concrete Deck Slabs: Parametric Study,” ACI Struct. J., vol. 116, no. 4, Jul. 2019, doi: 10.14359/51715565. View Article
[33] C09 Committee, “Test Method for Compressive Strength of Cylindrical Concrete Specimens.” ASTM International. doi: 10.1520/C0039_C0039M-18. View Article
[34] S. Yehia, A. Abdelfatah, and D. Mansour, “Effect of Aggregate Type and Specimen Configuration on Concrete Compressive Strength,” Crystals, vol. 10, no. 7, p. 625, Jul. 2020, doi: 10.3390/cryst10070625. View Article
[35] A. H. Buller, Mahboob Oad, and B. A. Memon, “Relationship between Cubical and Cylindrical Compressive Strength of Recycled Aggregate Concrete.” Unpublished, 2018. doi: 10.13140/RG.2.2.28872.78086.
[36] B. A. Memon, M. Oad, A. H. Buller, S. A. Shar, A. S. Buller, and F.-R. Abro, “Effect of Mould Size on Compressive Strength of Green Concrete Cubes,” Civ. Eng. J., vol. 5, no. 5, pp. 1181–1188, May 2019, doi: 10.28991/cej-2019-03091322. View Article
[37] A. H. Buller, A. Memon, Jahanzeb Sodhar, Mahboob Oad, and Irum Naz Sodhar, “EFFECT OF SPECIMEN SIZE ON STRENGTH OF GREEN CONCRETE CYLINDERS.” Unpublished, 2020. doi: 10.13140/RG.2.2.36300.77442.
[38] M. Batikha, S. T. M. Ali, A. Rostami, and M. Kurtayev, “Using recycled coarse aggregate and ceramic waste to produce sustainable economic concrete,” Int. J. Sustain. Eng., vol. 14, no. 4, pp. 785–799, Jul. 2021, doi: 10.1080/19397038.2020.1862353. View Article
[39] S. Amir, “Compressive Membrane Action in Prestressed Concrete Deck Slabs,” PhD thesis, Delft University of Technology, June 2014. View Article