Volume 5 - Year 2022- Pages 51-59
DOI: 10.11159/ijci.2022.008
A Review of Geotechnical Instabilities Identification and Monitoring At Deep Underground Mines: Case of Draa Sfar Mine in Morocco
Kaoutar Clero1,2, Said Ed-Diny2, Mouhamed Cherkaoui2, Tarik Soror3, Said Rziki3, Mohammed Achalhi2, Sanaa El Fkihi4, Andronic Boanarijesy1
1Moroccan Foundation for Advanced Science, Innovation and Research (MASCIR)
Mohamed Al Jazouli Street, Madinat Al Irfane, Rabat, Morocco
2National School of Mines of Rabat (ENSMR)
Hadj Ahmed Cherkaoui Avenue, Agdal, Rabat, Morocco
k.clero@mascir.ma; eddiny@enim.ac.ma
3REMINEX Mines et Carrières
BP 469, Marrakech, Morocco
4National School of Computer Science and Systems Analysis (ENSIAS), IRDA Team
Rabat IT Center, ADMIR Lab, Université Mohammed V, Rabat, Morocco
Abstract - This paper aims presenting a review about the identification of underground geotechnical instabilities and the analysis of the rock mass monitoring data at deep underground mines, and their application to the case study of Draa Sfar mine in Morocco. In fact, geotechnical conditions complexity increases in the mine’s deeper levels. Therefore, rock mass behaviour analysis, on-site geotechnical instrumentation and continuous monitoring of the medium are strongly required to avoid geotechnical risks. In this context, this paper focuses on presenting, first, a general state of the art about the most frequent rock mass instabilities encountered in the underground media, their classification, and their monitoring methods. In the following section, this paper provides general information about Draa Sfar mine and presents its geotechnical monitoring data analysis. At the end, conclusions about the current monitoring results and perspectives about innovative instrumentation solutions are discussed.
Keywords: Geotechnical instabilities - Underground mining – Rock mass behaviour - Geotechnical monitoring - Data analysis.
© Copyright 2022 Authors - This is an Open Access article published under the Creative Commons Attribution License terms. Unrestricted use, distribution, and reproduction in any medium are permitted, provided the original work is properly cited.
Date Received: 2022-05-10
Date Accepted: 2022-05-20
Date Published: 2022-06-08
1. Introduction
The occurrence of geotechnical instabilities at deep underground mines is related, on one hand, to the characteristics of the rock mass, whose behaviour is related to the rock matrix rheology and the joint sets and their distribution around the excavations. On the other hand, the activation of geotechnical failure mechanisms is related to the soliciting on-site conditions, such as the stress augmentation at important depth, the hydrogeological conditions, and the excavating action itself that destabilizes the initial equilibrium of the medium. Therefore, there are many parameters that should be considered while choosing the monitoring system to use on-site to anticipate the risks related to rock mass failure mechanisms at underground mines.
At Draa Sfar mine in Morocco, different lithologies and joint sets are encountered. Indeed, some formations at Draa Sfar mine are fractured and some facies are geomechanically weak. Besides, the current mining depth exceeds 1000m, which creates a high in-situ stress medium, requiring continuous monitoring of the mine to avoid geotechnical risks.
In this context, an instrumentation strategy was adopted in the mine since 2016. The analysis of the related monitoring data allows a better understanding of the rock mass behaviour on-site. This helps anticipating geotechnical risks and updating the monitoring and the supporting methods if needed.
2. State of the Art about Geotechnical Instabilities Classification and Monitoring in Underground Mines
2.1. Geotechnical instabilities classification in underground mines
Many rock instabilities can be encountered in underground mines. The main factors that lead to these instabilities are the stress augmentation with depth, the presence of rock mass discontinuities, and the water infiltration [1]. In fact, the stress augmentation can lead to the rock plastification or to the squeezing rocks phenomenon [2], which is a deformational behaviour of rocks that takes place slowly and gradually over time, such as buckling, especially in ductile rocks, as shown in Figure 1. On the other hand, the stress augmentation can lead to rock burst, which is a sudden and violent failure of rock, which occurs in high stress areas and especially in brittle rocks and that can be assimilated to a sudden explosion of the rock [3].
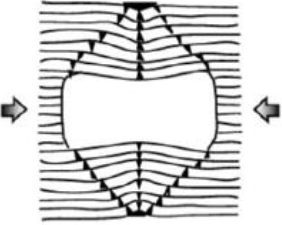
Besides, the presence of rock mass discontinuities with a developed network where many joint sets intersect, leads often to rock fall, especially if it is emphasized by other factors such as the hydrogeological factor. Rock mass discontinuities may lead also to rock bolts plastification and failure [4]. In fact, reinforcement bolts are designed to support tensile stress. However, once these bolts cross fractures, shear loads are generated and lead to the transition from bolts elastic behaviour to the plastic one, as represented in Figure 2. This plastification leads to the rock bolts failure over time.
Bolts failure can also be related to their corrosion [1]. In fact, before excavating, the ore body is in equilibrium with the surrounding rock in their geological environment. Once excavated, they become in contact with the humidity and oxygen of the air. This action creates a corrosive environment that leads to rock bolts corrosion and failure, and this phenomenon can be more emphasized by water infiltration through fractures.
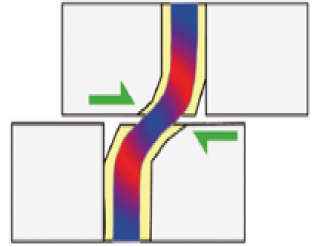
Besides, the creation of an excavation disturbs the equilibrium of the stress state. In fact, the intensity of the stress carried by the rock removed when the opening is formed is redistributed on other areas of the rock mass around the opening. This redistribution of stress can cause its increase in some regions and decrease in others, which leads to stress concentration phenomenon that can lead to rock failure in many cases.
Thus, regarding the different geotechnical instabilities present at the underground medium and considering different ways of their analysis in the literature [5][6], we suggest classifying them, such as presented in the chart in Figure 3, into:
- Progressive instabilities: which take time to occur progressively, such as the deformational behaviour of the rock mass by squeezing (buckling for example), and the rock plastification when the load exceeds the yield limit.
- Instantaneous instabilities: which occur suddenly and in short time, such as rock burst and rock fall. These instabilities are mainly related to brittle behaviour of rocks.
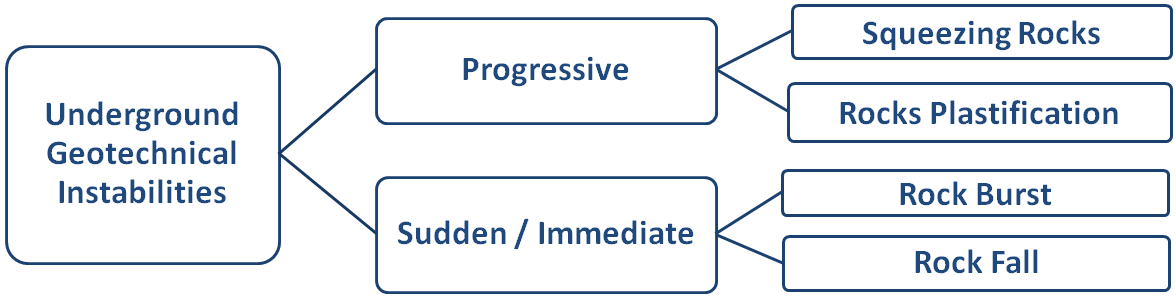
2.2. Geotechnical instabilities monitoring in underground mines
Starting from the suggested underground geotechnical instabilities classification, we can present their monitoring methods or tools through the same scheme, although it is not exhaustive. Besides, a review about the evolution of classical monitoring methods into innovative new methods will be emphasized. In fact, on one hand, progressive instabilities can be characterized through displacement, strain, or stress monitoring on the excavation sides or those of the reinforcement bolts. On the other hand, within instantaneous instabilities occurrence, such as rock burst or rock fall, the stored energy in the rock mass is suddenly released as a failure [7]. This action generates specific waves that can be monitored through specific methods.
2.2.1. Geotechnical monitoring methods for progressive instabilities
- Displacement’s monitoring
To monitor the excavation’s sides, extensometers and contractometers can be used, as shown in Figure 4. Extensometers allow measuring the distance between a head fixed on an excavation side and an anchor fixed deeper into the rock mass. Therefore, they measure relative displacements regarding a point which is supposed to be stationary over time. On the other hand, contractometers have two heads fixed on the excavation sides, in order to measure the convergence or divergence of the excavation [8].
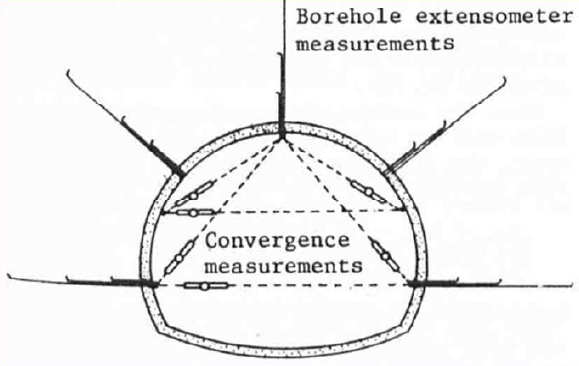
Classical extensometers and contractometers recently evolved into intelligent tools such as multi-point borehole extensometers (MPBX) and smart contractometers, which have embedded micro-controllers in their heads, and which incorporate digital signal processing that can be read through data loggers. Besides, the monitoring can be done wirelessly [9].
- Stress monitoring
The stress was monitored classically through traditional tests such as hydraulic fracturing, which is based on injecting pressurised water in a borehole while increasing its pressure until a new rock fracture is generated, then decreasing it until the new fracture get closed. This operation allows determining the primary stress state which is assimilated to the fracture closing pressure. This method is destructive, because it changes the excavation’s natural state to carry out the test [10].
Nowadays, stress gauges are used. We can present as an example, stress gauges based on vibrating wire sensors, which link the on-site stress with the magnitude of the wire tension and therefore to the change of natural vibration frequency, as shown in Figure 5. Therefore, monitoring the vibration allows identifying the stress state. Stress gauges can be also based on optical fibre sensors, where the reflected light wavelength changes when the stress changes. Thus, monitoring the wavelength variations allows identifying the stress state change [11].
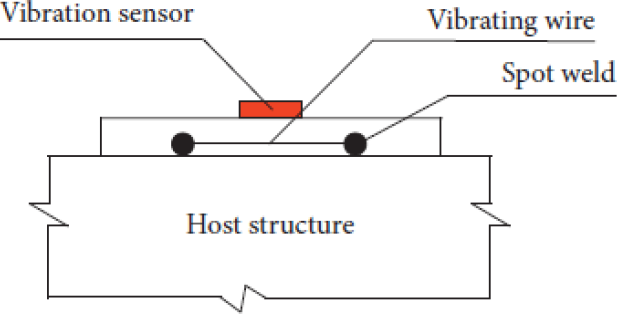
- Rock bolts’ monitoring
Rock bolts are used to reinforce and support the excavation sides against failure. Classical rock bolts can be classified into [12]:
- Mechanically anchored bolts, which are bonded to the rock mass just at the level of a shell. The main idea of this traditional method is to insert the bolt into the rock mass and then to rotate its end to force the shell to expand against the rock wall of the borehole.
- Friction anchored rock bolts, which are functioning through the frictional resistance generated from the interface along the whole bolt length that is in contact with the rock wall of the borehole.
- Fully grouted rock bolts, where the whole length of the bolt is bonded to the rock mass through resin or cement to ensure a better adhesion and avoid corrosion.
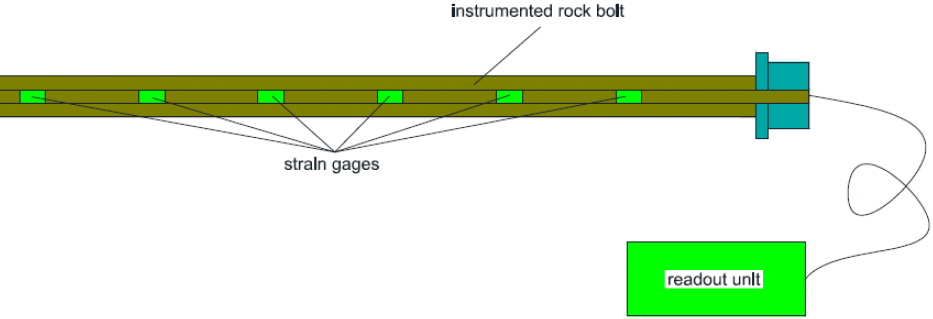
Besides, one of the main technologies that were developed recently in the field of rock bolts monitoring is called "the Cavimeter" [13]. This new technology, presented in Figure 7, consists of injecting, from a gas container embedded in the instrument, a pressurised gas in a plastic tube close to the rock bolt, and then monitoring its pressure variation through an interactive user interface. The pressure curve shape indicates if there are fractures or micro-cracks in the area where the bolt is installed, as shown in Figure 8. For instance, if the pressure is steady over time, there are neither fractures nor micro-cracks. Second, if the pressure curve slopes steeply and then stabilizes, there are fractures around the bolt installation area. Third, if the curve inclines with a weak slope without stabilizing, micro-cracks are present in the medium.
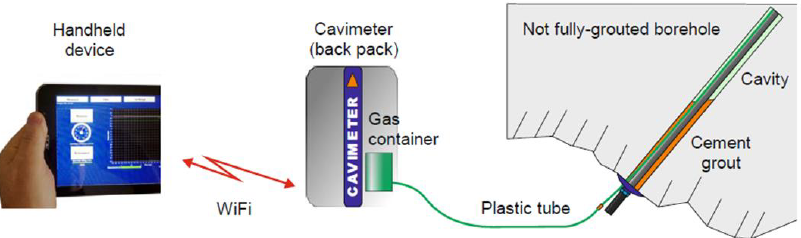
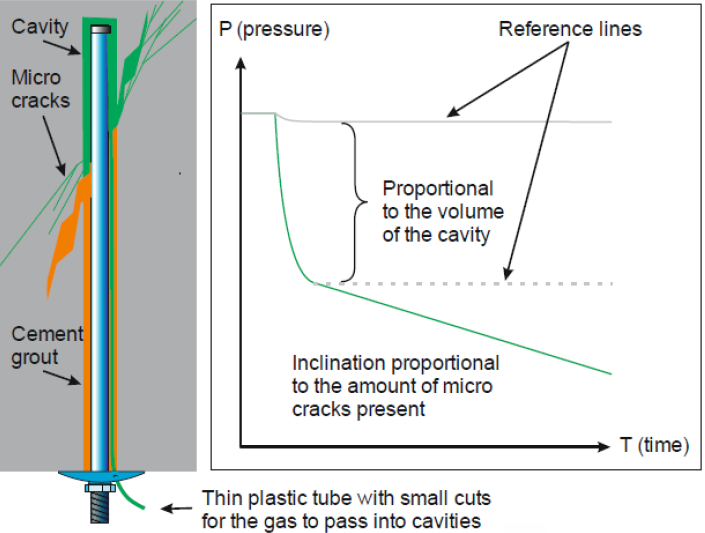
2.2.2. Geotechnical monitoring methods for instantaneous instabilities
As mentioned above, within instantaneous instabilities (e.g. block falls and rock bursts), the stored energy in the rock mass is suddenly released as a failure and generate therefore waves that can be monitored through seismic and acoustic recordings [7]. Seismic recording is mainly used in deep mines. There, the failure is mainly linked to the rock mass portions that have reached their resistance limit under the load of the overlying ground. Therefore, seismic waves are generated and can be intercepted by sensors such as seismometers, geophones, or accelerometers [14].
On the other hand, in shallow cavities, the overlying ground weight has a weak influence on the failure occurrence. There, rock failure is mainly linked to the alteration and fracturing effects. Instabilities such as block detachments and falls, breaks and cracks generate therefore acoustic waves, which propagate in the air and can be detected by microphones [15].
Nowadays, a new technology is used to detect instantaneous instabilities especially rock falls. Slough-meters, which are intelligent instruments, allow detecting any sloughing or breaking within the rock mass. In fact, Sloughmeters are formed by nodes embedded in the rock mass, each node forming a loop that transmits electric current. Sloughmeters contain also Light-Emitting Diodes (LED), each diode is linked to a current node. Therefore, the loss of a node, which is linked to a rock fall of the concerned block, is indicated by the absence of current in the loop and the LED switching off [16].
3. Case Study of Draa Sfar Mine in Morocco
3.1. General information about the mine
Draa Sfar is an underground mine in Morocco, producing primary metals (Cu, Zn, Pb). It is situated at 16 km on the Northwest of Marrakech city, as shown in Figure 9. Draa Sfar sector is subdivided into two sub-domains which are Draa Sfar North (Sidi Mbarek) and Draa Sfar South (Koudiat Tazakourt), and which are separated by the Tansifet River.
Draa Sfar North deposit is characterised by a well-developed iron cap on sedimentary facies, often masked by plio-quaternary alluvium, while the South side, which is concerned by the mining activity, is characterized by the presence of the main ore body, as well as most of the acidic volcanic formations and associated pyroclastic elements [17].
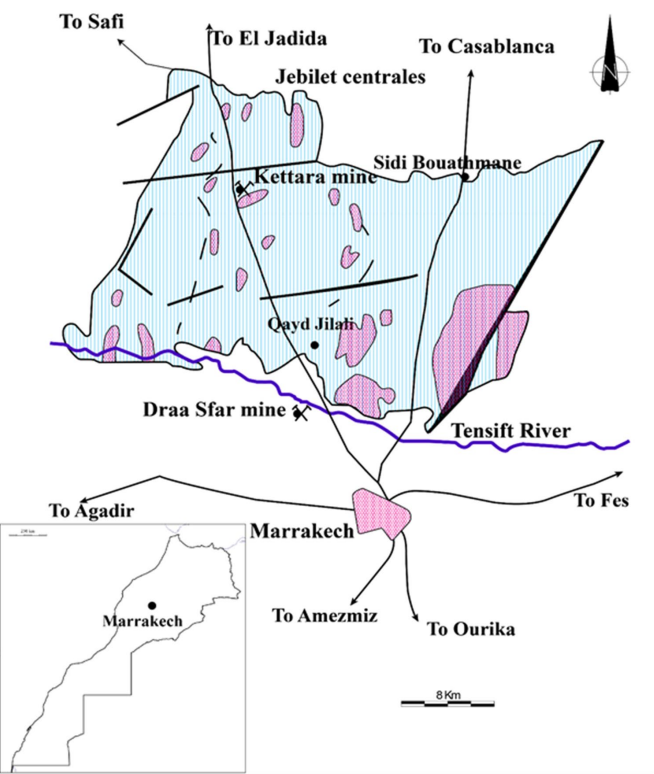
- Lithostratigraphy of the mine
Many surveys have been done by Draa Sfar mine’s geological service to establish a synthetic lithostratigraphic column, as represented in Figure 10. The following units are distinguished [19], and Table 1 shows their main characteristics:
- Rhyodacite igneous rock.
- Sandstone pelites and shales.
- Pyroclastic meta-tuffs with rhyodacite elements.
- Polymetallic massive sulphide ore body.
- Shales, black and calcareous pelites.
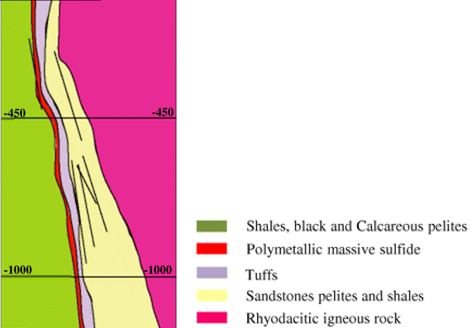
Table 1. Intact rocks characteristics at Draa Sfar mine.
Lithology |
UCS (MPa) |
Young Modulus E (GPa) |
Cohesion C (MPa) |
Friction Angle ϕ (°) |
Black Pelite |
30 |
10 |
1.7 |
30 |
Ore |
100 |
25 |
3 |
40 |
Tuff |
30 |
15 |
2.4 |
37 |
Rhyolite |
60 |
18 |
5.2 |
42.5 |
Sandy Pelite |
15 |
8 |
0.7 |
26 |
*UCS: Uniaxial Compressive Strength
- Joint sets
Draa Sfar formations underwent a series of deformations, summarised in three stages of different intensity. We distinguish the DI compressive stage, represented by S1 schistosity and folding, the DII stage, represented by S2 schistosity, and the DIII brittle stage, represented by NNW faults [17]. The major joint sets that have an important influence on the excavation’s stability study are represented with their main characteristics, in Table 2.
Table 2. Main joint sets characteristics at Draa Sfar mine.
Major joint set |
Dip (°) |
Dip Direction (°) |
Schistosity S1 |
80 |
100 |
Joint J1 (Sub-vertical) |
0 |
000 |
Joint J2 (Sub-horizontal) |
75 |
000 |
3.2. Instrumentation stations and data collection
To identify the rock mass behaviour and to assess the geotechnical instabilities at Draa Sfar mine, REMINEX installed instrumentation stations at the mine’s deep levels. In this section, we will focus on the extensometers installed at two stations, which are located respectively in the pelites and in the orebody. We focus on the identification of these two formations behaviour because, on one hand, pelites are the most dominating formation at the mine’s deeper levels as Figure 10 shows, and on the other hand, the ore body is the most concerned by the mining activity and its geotechnical response should be assessed.
The first station is located at –1000m level and contains an extensometer that measures the displacements of the pelites around the silo N°8. The second station is located at the intersection between the mineralization and the ramp of the –840 m level and contains an extensometer that measures the displacements in the orebody next to the ramp.
The data collection begins in February 2016. Thus, we will analyse the data of the three first months of instrumentation, which corresponds to the processing of 2160 measurements. In fact, for each station, a data logger is installed to collect measurements by a frequency of 1 hour in a continuous way. Raw data presents the measurement date and time, the instrument reference, the temperature, and the displacement values for each measuring anchor. There are six anchors in each extensometer installed at Draa Sfar (Multi-Point Borehole Extensometer).
3.3. Instrumentation data pre-processing
The displacements measurements that are collected are absolute temporally and spatially. To obtain the relative values, which are more interesting to analyse, and which are the basis of the establishment of the temporal graphs, equations (1) and (2) are used. Equation (1) allows obtaining the displacement’s variation for each anchor between the measurement time and the initial time, corresponding to the first measurement. Equation (2) allows to obtain the relative displacement’s variation between each anchor and the 6th one, which is considered as a reference as shown in Figure 11.


Where:
- Xri(t) is the temporal relative measurement for the anchor i at the instant t.
- Xai(t) is the absolute measurement for the anchor i at the instant t.
- Xai(t=0) is the initial absolute measurement for the anchor i at the beginning of the measuring period.
- Xrri(t) is the temporal and spatial relative measurement for the anchor i at the instant t, regarding the anchor 6.
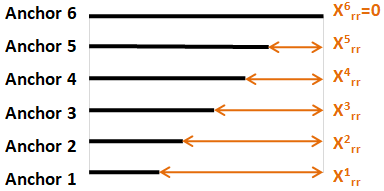
3.4. Instrumentation data analysis and results discussion
To have a better understanding of the collected data, and after the data pre-processing step, temporal graphs that show the variation of anchors displacements over the time were established, as shown in Figure 12 and Figure 14.
- First station’s extensometer
The temporal graph of this extensometer, which is installed in the pelites, presents a similar shape of the different anchors’ curves, even if reached values are different, as shown in Figure 12. The common shape consists of a low variation at the beginning, followed by a displacement peak, then a global stabilisation of the curves with little fluctuations for the remaining measurement period. This shape refers to a deformational time-dependent behaviour, which often characterizes elasto-visco-plastic materials having a ductile behaviour. In fact, considering a stress state that is relatively constant within the measuring period, we can confirm that the pelites present buckling creep behaviour. This is supported also by the visualization of these formations especially in the galleries whose direction is parallel to the foliation, as shown in Figure 13.
Concerning the difference of the reached values, it can be explained by the difference of the buckling potential locally in the rock mass, which gives to each anchor specific values for the displacement peak and the steady state value.
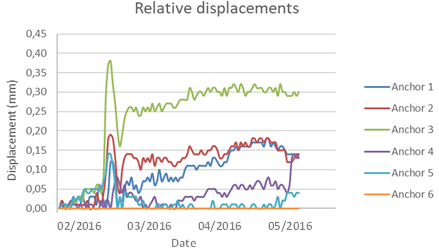
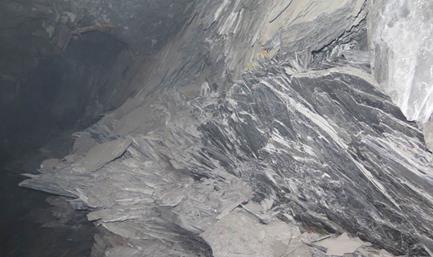
- Second station’s extensometer
The temporal graph of this extensometer, which is installed in the ore body, presents a similar shape of the different anchors’ curves, which have a monotonous variation increasing progressively with a relatively constant slope, as shown in Figure 14. This refers to the progressive development of cracks in the ore body. In fact, the mineralized formation is a competent one which presents a high compressive strength. Thus, it doesn’t present a deformational behaviour. Indeed, the ore body presents a brittle response that is characterized by cracks development progressively.
Concerning the peak observed on the first anchor’s curve, it refers to a rock block movement. In fact, the intersection of joints having different directions can create isolated blocks that are usually stabilized through supporting systems, such as rock bolts.
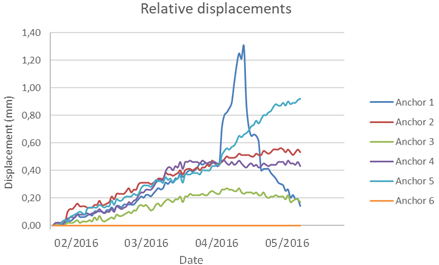
4. Conclusion
Many geotechnical instabilities can be encountered at underground mines. They concern weak rock masses having a deformational response, as well as the competent ones developing fracturing when affected by high stress or joint sets intersection. At Draa Sfar mine, the current excavation levels are below 1000m of depth, where the most dominant lithology is formed by pelites. Considering the mechanical characteristics of this formation, we can predict its deformational behaviour through creep buckling especially in the galleries whose direction is parallel to the foliation. This is confirmed also by the displacement graph of the first station’s extensometer. On the other hand, the displacement’s graph of the second station’s extensometer, installed in the ore body, shows the progressive development of fracturing in this competent formation. Therefore, the current instrumentation at Draa Sfar mine allows confirming the behaviour of the rock mass on-site, and to predict the corresponding eventual instabilities that may occur. However, this behaviour identification is done locally around each instrument’s installation area, which cannot cover the whole mine; besides, it consists of stationary measurement tools that are installed in a defined area once and for all. To overcome these limitations, and to prevent and anticipate risks related to geotechnical conditions on a large scale, the mining company CMG/Draa Sfar and REMINEX aim to develop innovative technical tools for dynamic auscultation of the whole mine. Therefore, the perspective of our research is to develop intelligent tools that allow detecting and preventing underground instabilities before they occur in different areas in the mine, and that will help to control the excavations stability through IoTs and artificial intelligence in a large and dynamic way.
5. Acknowledgements
We would like to thank the MANAGEM Group and its subsidiary CMG for allowing conducting research on the Draa Sfar site as an industrial partner in this project. As a reminder, this publication is part of the work undertaken by different partners composed of MASCIR (Moroccan Foundation for Advanced Science, Innovation and Research), REMINEX R&D and Engineering subsidiary of MANAGEM group, ENSMR (National School of Mines of Rabat), ENSIAS (National School of Computer Science and Systems Analysis) and UCA (University Cadi Ayyad). This research is conducted within the framework of the "Intelligent Connected Mine" project, which has been supported by the Moroccan Ministry of Higher Education, Scientific Research and Innovation, the Digital Development Agency (DDA), the National Center for Scientific and Technical Research of Morocco (CNRST) through the Al-Khawarizmi project in addition to MANAGEM group and MASCIR supports for this project.
References
[1] A. Jarni, M. Mouguina, A. Outzourith, M. Khadiri, S. Rziki and B. Imzilen, "Effets de l’environnement géologique et de la corrosion des boulons de soutènement sur la stabilité des ouvrages miniers: Cas du gisement polymétallique Draa Sfar (Jebilets Centrales, Maroc)" in Proceedings of the 8th international conference on magmatism, metamorphism, and associated mineralization, Marrakech, Morocco, 2013, pp. 9.
[2] S. Hammoum, "Modélisation numérique du comportement mécanique d'une excavation à grande profondeur à l'aide d'une loi d'écrouissage tenant compte des effets du temps-application à la mine Westwood". Ph.D. dissertation. Polytechnique School, Montreal, Canada, 2017.
[3] C. C. Li, P. Mikula, B. Simser, B. Hebblewhite, W. Joughin, X. Feng and N. Xu, "Discussions on rock burst and dynamic ground support in deep mines." Journal of Rock Mechanics and Geotechnical Engineering, vol. 11, no 5, p. 1110-1118, 2019. View Article
[4] CEREMA,"Protection contre les instabilités rocheuses: Dimensionnement et exécution des boulons", Methodological guide, Centre d'Etudes et d'expertise sur les Risques, l'Environnement, la Mobilité et l'Aménagement, 2017.
[5] W. Schubertand K. Grossauer, "Evaluation and interpretation of displacements in tunnels." in Proceedings of 14th International Conference on Engineering Surveying, Zürich, Switzerland, 2004.
[6] G. Ulivieri, S. Vezzosi, P. Farina and L. Meier, "On the use of acoustic records for the automatic detection and early warning of rock falls." in Proceedings of the 2020 International Symposium on Slope Stability in Open Pit Mining and Civil Engineering, Australian Centre for Geomechanics, Australia, 2020, p. 1193-1202. View Article
[7] P. K. Kaiser and M. Cai, "Design of rock support system under rockburst condition." Journal of Rock Mechanics and Geotechnical Engineering. vol. 4, no 3, p. 215-227, 2012. View Article
[8] S. Sakurai, "Interpretation of Displacement Measurements in Tunnels." in Proceedings of the ISRM International Symposium, Tokyo, Japan, OnePetro, 1981.
[9] A. J. Hyett, "Innovative Digital Instrumentation for Geotechnical Monitoring Systems." in Proceedings of the 2nd International Symposium of Narrow Vein Deposits, Val d’Or, Quebec, CIM, 2004.
[10] D. S. Subrahmanyam, G. Shyam, K. Vamshidhar and S. Vikram. "Hydraulic Fracturing Stress Measurements in Porous Rock Mass" in Proceedings of the ISRM International Symposium-10th Asian Rock Mechanics Symposium, Singapore, 2018.
[11] L. Wang, S. Xu, J. Qiu, K. Wang, E. Ma, C. Li, and C. Guo, "Automatic monitoring system in underground engineering construction: review and prospect". Advances in Civil Engineering, vol. 2020, 2020. View Article
[12] G. Song, W. Li, B. Wang and S. C. M. Ho, "A review of rock bolt monitoring using smart sensors". Sensors, pp 17(4), 776, 2017. View Article
[13] L.K.K.A. Gustafsson, “Sensor techniques to monitor installation and status of rock bolts” in Proceedings of the Eighth International Symposium on Ground Support in Mining and Underground Construction: “Ground Support 2016”, Kulturens Hus, Luleå, Sweden, pp. 1–13, 2016.
[14] A. Tonnellier, "Ecoute sismique des glissements de terrain dans les roches argilo-marneuses : détection et identification des sources intervenant dans la progression des glissements". Ph.D. dissertation, Strasbourg University, 2012.
[15] C. Bouffier, M. Bennani and P. Bigarre, "Surveillance du risque d’instabilité dans les cavités superficielles par méthode acoustique" in Proceedings of the National Days of Geotechnics and Engineering Geology (JNCG 2016), 2016.
[16] J. G. Henning, "Stability and access implications of open pit mining through old underground mine workings" in Proceedings of the 3rd International Symposium on Mine Safety Science and Engineering, pp. 165-169, 2016.
[17] S. Rziki,"Environnement géologique et modèle 3D du gisement polymétallique de Draa Sfar (Massif hercynien des Jebilets, Maroc): Implications et perspectives de développement". Ph.D. dissertation, Semlalia Faculty, Marrakech, Morocco, 2012.
[18] A., Boujghad, A. Bouabdli, and B. Baghdad, “Groundwater quality evaluation in the vicinity of the Draa Sfar Mine in Marrakesh, Morocco”. Euro-Mediterranean Journal for Environmental Integration, 4(1), 1-10, 2019. View Article
[19]L. Salama, E. M. Mouguina, E. El Bachari, L. Rddad, M. Outhounjite, M. Essaoudi, L. Maacha and M. Zouhair,"Numerical heat and fluid flow modelling of the Hercynian Draa Sfar polymetallic (Zn–Pb–Cu) massive sulphide deposit, Central Jbilets, Morocco", Arabian Journal of Geosciences, 11(24), 1-19, 2018. View Article